Discover
Hemispherics
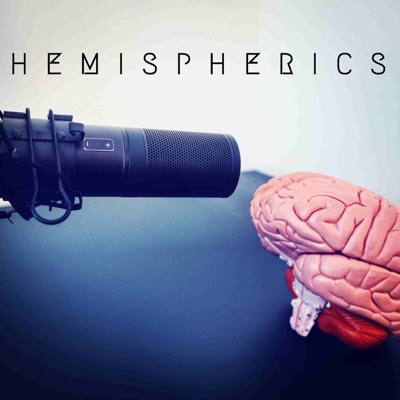
Hemispherics
Author: Hemispherics
Subscribed: 1Played: 24Subscribe
Share
© 2024 Hemispherics
Description
Hemispherics, el podcast de Fisioterapia y Neurorrehabilitación, presentado por Javier Sánchez Aguilar. En este podcast podrán encontrar:
• Reseñas de libros de neurociencia, neurorrehabilitación, fisioterapia.
• Comentarios de revisiones y artículos científicos relacionados con la fisioterapia y la neurorrehabilitación.
• Visibilización de investigadores/as.
• Exposición de temas específicos detallados sobre fisioterapia y neurorrehabilitación.
• Entrevistas a fisioterapeutas y especialistas en neurorrehabilitación.
• Reseñas de libros de neurociencia, neurorrehabilitación, fisioterapia.
• Comentarios de revisiones y artículos científicos relacionados con la fisioterapia y la neurorrehabilitación.
• Visibilización de investigadores/as.
• Exposición de temas específicos detallados sobre fisioterapia y neurorrehabilitación.
• Entrevistas a fisioterapeutas y especialistas en neurorrehabilitación.
71 Episodes
Reverse
En el episodio de hoy, os traigo un tema muy presente en neurorrehabilitación y en las consultas de neurología en relación con la esclerosis múltiple y es nada menos que la fatiga. La fatiga, ese síntoma tan temido desde siempre, tanto por pacientes como por profesionales de la salud, que es uno de los más reportados, con cifras de prevalencia entre 52 y el 90% de los pacientes (Nagaraj et al., 2013).
Indagamos en la fisiopatología de la fatiga para entender mejor este fenómeno, también diferentes formas de ver la fatiga con sus distintas nomenclaturas o términos, vamos a ver cómo se suele evaluar en el entorno clínico y en investigación y finalmente daremos algunas pinceladas de tratamiento neuromodulador.
Referencias del episodio:
1. Adibi, I., Sanayei, M., Tabibian, F., Ramezani, N., Pourmohammadi, A., & Azimzadeh, K. (2022). Multiple sclerosis-related fatigue lacks a unified definition: A narrative review. Journal of research in medical sciences : the official journal of Isfahan University of Medical Sciences, 27, 24. https://doi.org/10.4103/jrms.jrms_1401_20 (https://pubmed.ncbi.nlm.nih.gov/35419061/).
2. Ayache, S. S., & Chalah, M. A. (2017). Fatigue in multiple sclerosis - Insights into evaluation and management. Neurophysiologie clinique = Clinical neurophysiology, 47(2), 139–171. https://doi.org/10.1016/j.neucli.2017.02.004 (https://pubmed.ncbi.nlm.nih.gov/28416274/).
3. Ayache, S. S., Serratrice, N., Abi Lahoud, G. N., & Chalah, M. A. (2022). Fatigue in Multiple Sclerosis: A Review of the Exploratory and Therapeutic Potential of Non-Invasive Brain Stimulation. Frontiers in neurology, 13, 813965. https://doi.org/10.3389/fneur.2022.813965 (https://pubmed.ncbi.nlm.nih.gov/35572947/).
4. Bhattarai, J. J., Patel, K. S., Dunn, K. M., Brown, A., Opelt, B., & Hughes, A. J. (2023). Sleep disturbance and fatigue in multiple sclerosis: A systematic review and meta-analysis. Multiple sclerosis journal - experimental, translational and clinical, 9(3), 20552173231194352. https://doi.org/10.1177/20552173231194352 (https://pubmed.ncbi.nlm.nih.gov/37641617/).
5. Braley, T. J., & Chervin, R. D. (2010). Fatigue in multiple sclerosis: mechanisms, evaluation, and treatment. Sleep, 33(8), 1061–1067. https://doi.org/10.1093/sleep/33.8.1061 (https://www.ncbi.nlm.nih.gov/pmc/articles/PMC2910465/).
6. Capone, F., Motolese, F., Falato, E., Rossi, M., & Di Lazzaro, V. (2020). The Potential Role of Neurophysiology in the Management of Multiple Sclerosis-Related Fatigue. Frontiers in neurology, 11, 251. https://doi.org/10.3389/fneur.2020.00251 (https://www.ncbi.nlm.nih.gov/pmc/articles/PMC7212459/).
7. Chalah, M. A., Riachi, N., Ahdab, R., Créange, A., Lefaucheur, J. P., & Ayache, S. S. (2015). Fatigue in Multiple Sclerosis: Neural Correlates and the Role of Non-Invasive Brain Stimulation. Frontiers in cellular neuroscience, 9, 460. https://doi.org/10.3389/fncel.2015.00460 (https://pubmed.ncbi.nlm.nih.gov/26648845/).
8. Chalah, M. A., Kauv, P., Créange, A., Hodel, J., Lefaucheur, J. P., & Ayache, S. S. (2019). Neurophysiological, radiological and neuropsychological evaluation of fatigue in multiple sclerosis. Multiple sclerosis and related disorders, 28, 145–152. https://doi.org/10.1016/j.msard.2018.12.029 (https://pubmed.ncbi.nlm.nih.gov/30594815/).
9. Dittner, A. J., Wessely, S. C., & Brown, R. G. (2004). The assessment of fatigue: a practical guide for clinicians and researchers. Journal of psychosomatic research, 56(2), 157–170. https://doi.org/10.1016/S0022-3999(03)00371-4 (https://pubmed.ncbi.nlm.nih.gov/15016573/).
10. Dobryakova, E., Genova, H. M., DeLuca, J., & Wylie, G. R. (2015). The dopamine imbalance hypothesis of fatigue in multiple sclerosis and other neurological disorders. Frontiers in neurology, 6, 52. https://doi.org/10.3389/fneur.2015.00052 (https://pubmed.ncbi.nlm.nih.gov/25814977/).
11. Freal, J. E., Kraft, G. H., & Coryell, J. K. (1984). Symptomatic fatigue in multiple sclerosis. Archives of physical medicine and rehabilitation, 65(3), 135–138 (https://pubmed.ncbi.nlm.nih.gov/6703889/).
12. Gaede, G., Tiede, M., Lorenz, I., Brandt, A. U., Pfueller, C., Dörr, J., Bellmann-Strobl, J., Piper, S. K., Roth, Y., Zangen, A., Schippling, S., & Paul, F. (2017). Safety and preliminary efficacy of deep transcranial magnetic stimulation in MS-related fatigue. Neurology(R) neuroimmunology & neuroinflammation, 5(1), e423. https://doi.org/10.1212/NXI.0000000000000423 (https://pubmed.ncbi.nlm.nih.gov/29259998/).
13. Garis, G., Haupts, M., Duning, T., & Hildebrandt, H. (2023). Heart rate variability and fatigue in MS: two parallel pathways representing disseminated inflammatory processes?. Neurological sciences : official journal of the Italian Neurological Society and of the Italian Society of Clinical Neurophysiology, 44(1), 83–98. https://doi.org/10.1007/s10072-022-06385-1 (https://pubmed.ncbi.nlm.nih.gov/36125573/).
14. Hanken, K., Eling, P., & Hildebrandt, H. (2014). The representation of inflammatory signals in the brain - a model for subjective fatigue in multiple sclerosis. Frontiers in neurology, 5, 264. https://doi.org/10.3389/fneur.2014.00264 (https://pubmed.ncbi.nlm.nih.gov/25566171/).
15. Iriarte, J., Subirá, M. L., & Castro, P. (2000). Modalities of fatigue in multiple sclerosis: correlation with clinical and biological factors. Multiple sclerosis (Houndmills, Basingstoke, England), 6(2), 124–130. https://doi.org/10.1177/135245850000600212 (https://pubmed.ncbi.nlm.nih.gov/10773859/).
16. Jaeger, S., Paul, F., Scheel, M., Brandt, A., Heine, J., Pach, D., Witt, C. M., Bellmann-Strobl, J., & Finke, C. (2019). Multiple sclerosis-related fatigue: Altered resting-state functional connectivity of the ventral striatum and dorsolateral prefrontal cortex. Multiple sclerosis (Houndmills, Basingstoke, England), 25(4), 554–564. https://doi.org/10.1177/1352458518758911 (https://pubmed.ncbi.nlm.nih.gov/29464981/).
17. Langeskov-Christensen, M., Heine, M., Kwakkel, G., & Dalgas, U. (2015). Aerobic capacity in persons with multiple sclerosis: a systematic review and meta-analysis. Sports medicine (Auckland, N.Z.), 45(6), 905–923. https://doi.org/10.1007/s40279-015-0307-x (https://pubmed.ncbi.nlm.nih.gov/25739555/).
18. Linnhoff, S., Fiene, M., Heinze, H. J., & Zaehle, T. (2019). Cognitive Fatigue in Multiple Sclerosis: An Objective Approach to Diagnosis and Treatment by Transcranial Electrical Stimulation. Brain sciences, 9(5), 100. https://doi.org/10.3390/brainsci9050100 (https://pubmed.ncbi.nlm.nih.gov/31052593/).
19. Liu, M., Fan, S., Xu, Y., & Cui, L. (2019). Non-invasive brain stimulation for fatigue in multiple sclerosis patients: A systematic review and meta-analysis. Multiple sclerosis and related disorders, 36, 101375. https://doi.org/10.1016/j.msard.2019.08.017 (https://pubmed.ncbi.nlm.nih.gov/31491597/).
20. Loy, B. D., Taylor, R. L., Fling, B. W., & Horak, F. B. (2017). Relationship between perceived fatigue and performance fatigability in people with multiple sclerosis: A systematic review and meta-analysis. Journal of psychosomatic research, 100, 1–7. https://doi.org/10.1016/j.jpsychores.2017.06.017 (https://pubmed.ncbi.nlm.nih.gov/28789787/).
21. Mills, R. J., & Young, C. A. (2008). A medical definition of fatigue in multiple sclerosis. QJM : monthly journal of the Association of Physicians, 101(1), 49–60. https://doi.org/10.1093/qjmed/hcm122 (https://pubmed.ncbi.nlm.nih.gov/18194977/).
22. Nagaraj, K., Taly, A. B., Gupta, A., Prasad, C., & Christopher, R. (2013). Prevalence of fatigue in patients with multiple sclerosis and its effect on the quality of life. Journal of neurosciences in rural practice, 4(3), 278–282. https://doi.org/10.4103/0976-3147.118774 (https://www.ncbi.nlm.nih.gov/pmc/articles/PMC3821412/).
23. Patejdl, R., Penner, I. K., Noack, T. K., & Zettl, U. K. (2016). Multiple sclerosis and fatigue: A review on the contribution of inflammation and immune-mediated neurodegeneration. Autoimmunity reviews, 15(3), 210–220. https://doi.org/10.1016/j.autrev.2015.11.005 (https://pubmed.ncbi.nlm.nih.gov/26589194/).
24. Patejdl, R., & Zettl, U. K. (2022). The pathophysiology of motor fatigue and fatigability in multiple sclerosis. Frontiers in neurology, 13, 891415. https://doi.org/10.3389/fneur.2022.891415 (https://pubmed.ncbi.nlm.nih.gov/35968278/).
25. Torres-Costoso, A., Martínez-Vizcaíno, V., Reina-Gutiérrez, S., Álvarez-Bueno, C., Guzmán-Pavón, M. J., Pozuelo-Carrascosa, D. P., Fernández-Rodríguez, R., Sanchez-López, M., & Cavero-Redondo, I. (2022). Effect of Exercise on Fatigue in Multiple Sclerosis: A Network Meta-analysis Comparing Different Types of Exercise. Archives of physical medicine and rehabilitation, 103(5), 970–987.e18. https://doi.org/10.1016/j.apmr.2021.08.008 (https://pubmed.ncbi.nlm.nih.gov/34509464/).
26. Yang, T. T., Wang, L., Deng, X. Y., & Yu, G. (2017). Pharmacological treatments for fatigue in patients with multiple sclerosis: A systematic review and meta-analysis. Journal of the neurological sciences, 380, 256–261. https://doi.org/10.1016/j.jns.2017.07.042 (https://pubmed.ncbi.nlm.nih.gov/28870581/).
27. Zimek, D., Miklusova, M., & Mares, J. (2023). Overview of the Current Pathophysiology of Fatigue in Multiple Sclerosis, Its Diagnosis and Treatment Options - Review Article. Neuropsychiatric disease and treatment, 19, 2485–2497. https://doi.org/10.2147/NDT.S429862 (https://pubmed.ncbi.nlm.nih.gov/38029042/).
28. Zhou, X., Li, K., Chen, S., Zhou, W., Li, J., Huang, Q., Xu, T., Gao, Z., Wang, D., Zhao, S., & Dong, H. (2022). Clinical application of transcranial magnetic stimulation in multiple sclerosis. Frontiers in immunology, 13, 902658. https://doi.org/10.3389/fimmu.2022.902658 (https://www.ncbi.nlm.nih.gov/pmc/articles/PMC9483183/).
En este episodio, realizo una síntesis de conocimiento y experiencia sobre el cerebelo y la ataxia, desde la anatomía y fisiología, describiendo la ataxia, hasta la evaluación y el tratamiento neurorrehabilitador. El objetivo es hilar el conocimiento anatómico y neurofisiológico con el aprendizaje motor y la plasticidad del cerebelo y tratar de establecer un marco de evaluación y tratamiento de los pacientes atáxicos.
Referencias bibliográficas:
1. Stephan, M. A., et al (2011). Effect of long-term climbing training on cerebellar ataxia: a case series. Rehabilitation research and practice, 2011, 525879. (https://pubmed.ncbi.nlm.nih.gov/22191034/).
2. Aprigliano, F., et al (2019). Effects of repeated waist-pull perturbations on gait stability in subjects with cerebellar ataxia. Journal of neuroengineering and rehabilitation, 16(1), 50. (https://pubmed.ncbi.nlm.nih.gov/30975168/9.
3. Benussi, A.,et al (2017). Long term clinical and neurophysiological effects of cerebellar transcranial direct current stimulation in patients with neurodegenerative ataxia. Brain stimulation, 10(2), 242–250. (https://pubmed.ncbi.nlm.nih.gov/27838276/).
4. Bostan, A. C., & Strick, P. L. (2018). The basal ganglia and the cerebellum: nodes in an integrated network. Nature reviews. Neuroscience, 19(6), 338–350. (https://pubmed.ncbi.nlm.nih.gov/29643480/).
5. Cabaraux, P., et al (2023). Consensus Paper: Ataxic Gait. Cerebellum (London, England), 22(3), 394–430. (https://pubmed.ncbi.nlm.nih.gov/35414041/).
6. D'Angelo E. (2014). The organization of plasticity in the cerebellar cortex: from synapses to control. Progress in brain research, 210, 31–58. (https://pubmed.ncbi.nlm.nih.gov/24916288/).
7. D'Angelo E. (2018). Physiology of the cerebellum. Handbook of clinical neurology, 154, 85–108. (https://pubmed.ncbi.nlm.nih.gov/29903454/).
8. França, C., et al (2018). Effects of cerebellar neuromodulation in movement disorders: A systematic review. Brain stimulation, 11(2), 249–260. (https://pubmed.ncbi.nlm.nih.gov/29191439/).
9. Gong, C., et al (2023). Efficacy and safety of noninvasive brain stimulation for patients with cerebellar ataxia: a systematic review and meta-analysis of randomized controlled trials. Journal of neurology, 270(10), 4782–4799. (https://pubmed.ncbi.nlm.nih.gov/37460852/).
10. Gorgas, A. M., et al (2015). Gait changes with balance-based torso-weighting in people with multiple sclerosis. (https://pubmed.ncbi.nlm.nih.gov/24930996/).
11. Ilg, W., et al (2023). Quantitative Gait and Balance Outcomes for Ataxia Trials. Cerebellum 10.1007/s12311-023-01625-2. Advance online publication. (https://pubmed.ncbi.nlm.nih.gov/37955812/).
12. Ilg, W., et al (2009). Intensive coordinative training improves motor performance in degenerative cerebellar disease. Neurology, 73(22), 1823–1830. (https://pubmed.ncbi.nlm.nih.gov/19864636/).
13. Jacobson, G. A. et al (2008). A model of the olivo-cerebellar system as a temporal pattern generator. Trends in neurosciences, 31(12), 617–625. (https://pubmed.ncbi.nlm.nih.gov/18952303/).
14. Kelly, G., & Shanley, J. (2016). Rehabilitation of ataxic gait following cerebellar lesions: Applying theory to practice. Physiotherapy theory and practice, 32(6), 430–437. (https://pubmed.ncbi.nlm.nih.gov/27458875/).
15. Marsden J. F. (2018). Cerebellar ataxia. Handbook of clinical neurology, 159, 261–281. (https://pubmed.ncbi.nlm.nih.gov/30482319/).
16. Morton, S. M., & Bastian, A. J. (2003). Relative contributions of balance and voluntary leg-coordination deficits to cerebellar gait ataxia. Journal of neurophysiology, 89(4), 1844–1856. (https://pubmed.ncbi.nlm.nih.gov/12612041/).
17. Ruggieri, S., et al (2021). A matter of atrophy: differential impact of brain and spine damage on disability worsening in multiple sclerosis. Journal of neurology, 268(12), 4698–4706. (https://pubmed.ncbi.nlm.nih.gov/33942160/).
18. Serrao, M., et al (2017). Use of dynamic movement orthoses to improve gait stability and trunk control in ataxic patients. European journal of physical and rehabilitation medicine, 53(5), 735–743. (https://pubmed.ncbi.nlm.nih.gov/28627859/).
19. Shah, V. V., et al (2021). Gait Variability in Spinocerebellar Ataxia Assessed Using Wearable Inertial Sensors. Movement disorders : official journal of the Movement Disorder Society, 36(12), 2922–2931. (https://pubmed.ncbi.nlm.nih.gov/34424581/).
20. Wang, Y., et al (2023). Effects of transcranial magnetic stimulation on cerebellar ataxia: A systematic review and meta-analysis. Frontiers in neurology, 14, 1049813. (https://pubmed.ncbi.nlm.nih.gov/36779066/).
21. Wright, R. L., et al (2016). Metronome Cueing of Walking Reduces Gait Variability after a Cerebellar Stroke. Frontiers in neurology, 7, 84. (https://pubmed.ncbi.nlm.nih.gov/27313563/).
En este episodio, actualizamos la neuroanatomía funcional del núcleo rojo a raíz del último trabajo pre-print de Krimmel (2024): The brainstem’s red nucleus was evolutionarily upgraded to support goal-directed action. Aprovechamos para traer de vuelta la crítica al tracto rubroespinal que hicimos en el #1 de Hemispherics y argumentamos el sentido evolutivo y funcional del núcleo rojo y sus conexiones en el ser humano adulto.
¿Es el núcleo rojo motor? ¿O tiene más función como nodo en una red más amplia de control ejecutivo de la acción? ¡Lo vemos en este episodio!
Referencias del episodio:
1. Habas, C., & Cabanis, E. A. (2006). Cortical projections to the human red nucleus: a diffusion tensor tractography study with a 1.5-T MRI machine. Neuroradiology, 48(10), 755–762. https://doi.org/10.1007/s00234-006-0117-9 (https://pubmed.ncbi.nlm.nih.gov/16937147/).
2. Basile, G. A., Quartu, M., Bertino, S., Serra, M. P., Boi, M., Bramanti, A., Anastasi, G. P., Milardi, D., & Cacciola, A. (2021). Red nucleus structure and function: from anatomy to clinical neurosciences. Brain structure & function, 226(1), 69–91. https://doi.org/10.1007/s00429-020-02171-x (https://pubmed.ncbi.nlm.nih.gov/33180142/).
3. Sung, Y. W., Kiyama, S., Choi, U. S., & Ogawa, S. (2022). Involvement of the intrinsic functional network of the red nucleus in complex behavioral processing. Cerebral cortex communications, 3(3), tgac037. https://doi.org/10.1093/texcom/tgac037 (https://www.ncbi.nlm.nih.gov/pmc/articles/PMC9491841/).
4. Krimmel, S.R., et al. (2024). The brainstem’s red nucleus was evolutionarily upgraded to support goal-directed action. bioRxiv. Preprint. https://doi.org/10.1101/2023.12.30.573730 (https://www.biorxiv.org/content/10.1101/2023.12.30.573730v1).
5. Gordon, E. M., Chauvin, R. J., Van, A. N., Rajesh, A., Nielsen, A., Newbold, D. J., Lynch, C. J., Seider, N. A., Krimmel, S. R., Scheidter, K. M., Monk, J., Miller, R. L., Metoki, A., Montez, D. F., Zheng, A., Elbau, I., Madison, T., Nishino, T., Myers, M. J., Kaplan, S., … Dosenbach, N. U. F. (2023). A somato-cognitive action network alternates with effector regions in motor cortex. Nature, 617(7960), 351–359. https://doi.org/10.1038/s41586-023-05964-2 (https://pubmed.ncbi.nlm.nih.gov/37076628/).
En este episodio, resumo un artículo reciente (2023) de John Krakauer y Tamar Makin sobre la reorganización cortical. Es un artículo crítica a este concepto tan famoso y vanagloriado en neurociencia y se aportan datos que apoyan esta crítica mencionando estudios clásicos en animales y humanos. Estudios sobre ceguera congénita, experimentos en gatos y hurones, amputados y reorganización tras un ictus. Krakauer propone su definición y criterios de reorganización cortical y en base a eso refuta los estudios que afirman la reorganización.
Referencias del episodio:
1. Makin, T. R., & Krakauer, J. W. (2023). Against cortical reorganisation. eLife, 12, e84716. https://doi.org/10.7554/eLife.84716 (https://pubmed.ncbi.nlm.nih.gov/37986628/).
2. Kilgard, M. P., & Merzenich, M. M. (1998). Cortical map reorganization enabled by nucleus basalis activity. Science (New York, N.Y.), 279(5357), 1714–1718. https://doi.org/10.1126/science.279.5357.1714 (https://pubmed.ncbi.nlm.nih.gov/9497289/).
3. Pascual-Leone, A., & Torres, F. (1993). Plasticity of the sensorimotor cortex representation of the reading finger in Braille readers. Brain : a journal of neurology, 116 ( Pt 1), 39–52. https://doi.org/10.1093/brain/116.1.39 (https://pubmed.ncbi.nlm.nih.gov/8453464/).
4. Nudo R. J. (2007). Postinfarct cortical plasticity and behavioral recovery. Stroke, 38(2 Suppl), 840–845. https://doi.org/10.1161/01.STR.0000247943.12887.d2 (https://pubmed.ncbi.nlm.nih.gov/17261749/).
5. Ramachandran, V. S., Stewart, M., & Rogers-Ramachandran, D. C. (1992). Perceptual correlates of massive cortical reorganization. Neuroreport, 3(7), 583–586. https://doi.org/10.1097/00001756-199207000-00009 (https://pubmed.ncbi.nlm.nih.gov/1421112/).
6. Wiesel, T. N., & Hubel, D. H. (1963). Single-cell responses in striate cortex of kittens deprived of vision in one eye. Journal of neurophysiology, 26, 1003–1017. https://doi.org/10.1152/jn.1963.26.6.1003 (https://pubmed.ncbi.nlm.nih.gov/14084161/).
Este es un episodio muy especial, en el que resumo la biografía de Cajal y sus principales descubrimientos en neurociencia, además de hablar de su contexto y principales discípulos. Es un episodio homenaje a una figura muy poco reconocida en España para la relevancia que tiene en la neurociencia moderna. Cajal ganó el Premio Nobel en Fisiología y Medicina en 1906, compartido con Camillo Golgi, en reconocimiento a su “teoría neuronal” y a su investigación sobre histología del sistema nervioso del humano y los vertebrados. Obtuvo innumerables galardones, como la Medalla Helmholtz en 1905, Premio Nacional de Moscú en 1900 y Doctor Honoris Causa en muchas universidades.
Referencias del episodio:
1. Cánovas Sánchez F. (2021). Cajal. Alianza Editorial.
2. Swanson W. Larry & Newman Eric (2017). The Beautiful Brain: The Drawings of Santiago Ramon y Cajal. Abrams.
3.de Castro F. (2019). Cajal and the Spanish Neurological School: Neuroscience Would Have Been a Different Story Without Them. Frontiers in cellular neuroscience, 13, 187. https://doi.org/10.3389/fncel.2019.00187 (https://pubmed.ncbi.nlm.nih.gov/31178695/).
4. Alonso Peña Jose Ramón & De Carlos Segovia Juan Andrés (2018). Cajal: un grito por la ciencia. Next Door Publishers S.L.
En este episodio, hablo sobre vibración focal en neurorrehabilitación del adulto, apoyándome posteriormente en la charla con el fisioterapeuta Serafín Ortigueira, quien tiene amplia experiencia con esta técnica. Describo brevemente la vibración focal, sus mecanismos de acción y algunas de las aplicaciones clínicas actuales, sobre todo en pacientes con ictus y lesión medular.
La vibración focal es una técnica sencilla de aplicar, pero tiene mecanismos que deben ser comprendidos para aplicarla con toda su riqueza y posibilidades que brinda, ya sea para el tratamiento de la espasticidad, mejora del control motor o o incluso aspectos coaduyaventes a nivel visceral o respiratorio.
Referencias del episodio:
1. Shinohara M. (2005). Effects of prolonged vibration on motor unit activity and motor performance. Medicine and science in sports and exercise, 37(12), 2120–2125. https://doi.org/10.1249/01.mss.0000178106.68569.7e (https://pubmed.ncbi.nlm.nih.gov/16331139/).
2. Khalifeloo, M., Naghdi, S., Ansari, N. N., Akbari, M., Jalaie, S., Jannat, D., & Hasson, S. (2018). A study on the immediate effects of plantar vibration on balance dysfunction in patients with stroke. Journal of exercise rehabilitation, 14(2), 259–266. https://doi.org/10.12965/jer.1836044.022 (https://pubmed.ncbi.nlm.nih.gov/29740561/).
3. Karimi-AhmadAbadi, A., Naghdi, S., Ansari, N. N., Fakhari, Z., & Khalifeloo, M. (2018). A clinical single blind study to investigate the immediate effects of plantar vibration on balance in patients after stroke. Journal of bodywork and movement therapies, 22(2), 242–246. https://doi.org/10.1016/j.jbmt.2017.04.013 (https://pubmed.ncbi.nlm.nih.gov/29861214/).
4. Celletti, C., Suppa, A., Bianchini, E., Lakin, S., Toscano, M., La Torre, G., Di Piero, V., & Camerota, F. (2020). Promoting post-stroke recovery through focal or whole body vibration: criticisms and prospects from a narrative review. Neurological sciences : official journal of the Italian Neurological Society and of the Italian Society of Clinical Neurophysiology, 41(1), 11–24. https://doi.org/10.1007/s10072-019-04047-3 (https://pubmed.ncbi.nlm.nih.gov/31468237/).
5. Paoloni, M., Mangone, M., Scettri, P., Procaccianti, R., Cometa, A., & Santilli, V. (2010). Segmental muscle vibration improves walking in chronic stroke patients with foot drop: a randomized controlled trial. Neurorehabilitation and neural repair, 24(3), 254–262. https://doi.org/10.1177/1545968309349940 (https://pubmed.ncbi.nlm.nih.gov/19855076/).
6. Moggio, L., de Sire, A., Marotta, N., Demeco, A., & Ammendolia, A. (2022). Vibration therapy role in neurological diseases rehabilitation: an umbrella review of systematic reviews. Disability and rehabilitation, 44(20), 5741–5749. https://doi.org/10.1080/09638288.2021.1946175 (https://pubmed.ncbi.nlm.nih.gov/34225557/).
7. Rosenkranz, K., & Rothwell, J. C. (2003). Differential effect of muscle vibration on intracortical inhibitory circuits in humans. The Journal of physiology, 551(Pt 2), 649–660. https://doi.org/10.1113/jphysiol.2003.043752 (https://www.ncbi.nlm.nih.gov/pmc/articles/PMC2343209/).
8. Binder, C., Kaya, A. E., & Liepert, J. (2009). Vibration prolongs the cortical silent period in an antagonistic muscle. Muscle & nerve, 39(6), 776–780. https://doi.org/10.1002/mus.21240 (https://pubmed.ncbi.nlm.nih.gov/19334048/).
9. Bertasi, V., Bertolasi, L., Frasson, E., & Priori, A. (2000). The excitability of human cortical inhibitory circuits responsible for the muscle silent period after transcranial brain stimulation. Experimental brain research, 132(3), 384–389. https://doi.org/10.1007/s002210000352 (https://pubmed.ncbi.nlm.nih.gov/10883387/).
10. Mortaza, N., Abou-Setta, A. M., Zarychanski, R., Loewen, H., Rabbani, R., & Glazebrook, C. M. (2019). Upper limb tendon/muscle vibration in persons with subacute and chronic stroke: a systematic review and meta-analysis. European journal of physical and rehabilitation medicine, 55(5), 558–569. https://doi.org/10.23736/S1973-9087.19.05605-3 (https://pubmed.ncbi.nlm.nih.gov/30868835/).
11. Avvantaggiato, C., Casale, R., Cinone, N., Facciorusso, S., Turitto, A., Stuppiello, L., Picelli, A., Ranieri, M., Intiso, D., Fiore, P., Ciritella, C., & Santamato, A. (2021). Localized muscle vibration in the treatment of motor impairment and spasticity in post-stroke patients: a systematic review. European journal of physical and rehabilitation medicine, 57(1), 44–60. https://doi.org/10.23736/S1973-9087.20.06390-X (https://pubmed.ncbi.nlm.nih.gov/33111513/).
12. Murillo, N., Valls-Sole, J., Vidal, J., Opisso, E., Medina, J., & Kumru, H. (2014). Focal vibration in neurorehabilitation. European journal of physical and rehabilitation medicine, 50(2), 231–242 (https://pubmed.ncbi.nlm.nih.gov/24842220/).
13. Li, W., Luo, F., Xu, Q., Liu, A., Mo, L., Li, C., & Ji, L. (2022). Brain oscillatory activity correlates with the relief of post-stroke spasticity following focal vibration. Journal of integrative neuroscience, 21(3), 96. https://doi.org/10.31083/j.jin2103096 (https://pubmed.ncbi.nlm.nih.gov/35633177/).
14. Murillo, N., Kumru, H., Vidal-Samso, J., Benito, J., Medina, J., Navarro, X., & Valls-Sole, J. (2011). Decrease of spasticity with muscle vibration in patients with spinal cord injury. Clinical neurophysiology : official journal of the International Federation of Clinical Neurophysiology, 122(6), 1183–1189. https://doi.org/10.1016/j.clinph.2010.11.012 (https://pubmed.ncbi.nlm.nih.gov/21172739/).
15. Murillo, N. (2011). Neuromodulación de la espasticidad en pacientes con lesión medular mediante vibración y estimulación magnética transcraneal (http://hdl.handle.net/10803/3840).
16. Wang, H., Chandrashekhar, R., Rippetoe, J., & Ghazi, M. (2020). Focal Muscle Vibration for Stroke Rehabilitation: A Review of Vibration Parameters and Protocols. Applied Sciences, 10(22), 8270. MDPI AG. Retrieved from http://dx.doi.org/10.3390/app10228270 (https://www.mdpi.com/2076-3417/10/22/8270).
17. Filippi, G. M., Rodio, A., Fattorini, L., Faralli, M., Ricci, G., & Pettorossi, V. E. (2023). Plastic changes induced by muscle focal vibration: A possible mechanism for long-term motor improvements. Frontiers in neuroscience, 17, 1112232. https://doi.org/10.3389/fnins.2023.1112232 (https://www.ncbi.nlm.nih.gov/pmc/articles/PMC9992721/).
18. Hagbarth, K. E., & Eklund, G. (1969). The muscle vibrator--a useful tool in neurological therapeutic work. Scandinavian journal of rehabilitation medicine, 1(1), 26–34 (https://pubmed.ncbi.nlm.nih.gov/5406721/).
19. Zapatillas Nushu (https://magnes.ch/solutions/nushu/).
20. Sadeghi, M., & Sawatzky, B. (2014). Effects of vibration on spasticity in individuals with spinal cord injury: a scoping systematic review. American journal of physical medicine & rehabilitation, 93(11), 995–1007. https://doi.org/10.1097/PHM.0000000000000098 (https://pubmed.ncbi.nlm.nih.gov/24743464/).
21. DeForest, B. A., Bohorquez, J., & Perez, M. A. (2020). Vibration attenuates spasm-like activity in humans with spinal cord injury. The Journal of physiology, 598(13), 2703–2717. https://doi.org/10.1113/JP279478 (https://pubmed.ncbi.nlm.nih.gov/32298483/).
22. Calabrò, R. S., Naro, A., Russo, M., Milardi, D., Leo, A., Filoni, S., Trinchera, A., & Bramanti, P. (2017). Is two better than one? Muscle vibration plus robotic rehabilitation to improve upper limb spasticity and function: A pilot randomized controlled trial. PloS one, 12(10), e0185936. https://doi.org/10.1371/journal.pone.0185936 (https://pubmed.ncbi.nlm.nih.gov/28973024/).
23. Chen, Y. L., Jiang, L. J., Cheng, Y. Y., Chen, C., Hu, J., Zhang, A. J., Hua, Y., & Bai, Y. L. (2023). Focal vibration of the plantarflexor and dorsiflexor muscles improves poststroke spasticity: a randomized single-blind controlled trial. Annals of physical and rehabilitation medicine, 66(3), 101670. https://doi.org/10.1016/j.rehab.2022.101670 (https://pubmed.ncbi.nlm.nih.gov/35940478/).
En el episodio de hoy, tenemos delante un tema muy desconocido en neurorrehabilitación aunque muy relevante como es el sistema nervioso autónomo. Todos los profesionales de la salud y especialmente los que nos dedicamos a los pacientes neurológicos, tenemos una cierta base teórica sobre el sistema nervioso autónomo, si bien peca mucho de lo periférico, cuando existe una representación central (la red autónoma central) que ejerce control sobre el sistema autónomo y tiene implicaciones en patología neurológica, incluso en el tratamiento. Hablamos de variabilidad de frecuencia cardíaca como variable autónoma fundamental y de algunos modelos vagales cardíacos que explican la conexión cerebro-corazón.
Referencias del episodio:
1. Sposato, L. A., Hilz, M. J., Aspberg, S., Murthy, S. B., Bahit, M. C., Hsieh, C. Y., Sheppard, M. N., Scheitz, J. F., & World Stroke Organisation Brain & Heart Task Force (2020). Post-Stroke Cardiovascular Complications and Neurogenic Cardiac Injury: JACC State-of-the-Art Review. Journal of the American College of Cardiology, 76(23), 2768–2785. https://doi.org/10.1016/j.jacc.2020.10.009 (https://pubmed.ncbi.nlm.nih.gov/33272372/).
2. Porges S. W. (2009). The polyvagal theory: new insights into adaptive reactions of the autonomic nervous system. Cleveland Clinic journal of medicine, 76 Suppl 2(Suppl 2), S86–S90. https://doi.org/10.3949/ccjm.76.s2.17 (https://www.ncbi.nlm.nih.gov/pmc/articles/PMC3108032/).
3. Sletten, D. M., Suarez, G. A., Low, P. A., Mandrekar, J., & Singer, W. (2012). COMPASS 31: a refined and abbreviated Composite Autonomic Symptom Score. Mayo Clinic proceedings, 87(12), 1196–1201. https://doi.org/10.1016/j.mayocp.2012.10.013 (https://pubmed.ncbi.nlm.nih.gov/23218087/).
4. Nikolin, S., Boonstra, T. W., Loo, C. K., & Martin, D. (2017). Combined effect of prefrontal transcranial direct current stimulation and a working memory task on heart rate variability. PloS one, 12(8), e0181833. https://doi.org/10.1371/journal.pone.0181833 (https://pubmed.ncbi.nlm.nih.gov/28771509/).
5. Vistisen, S. T., Jensen, J., Fleischer, J., & Nielsen, J. F. (2015). Association between the sensory-motor nervous system and the autonomic nervous system in neurorehabilitation patients with severe acquired brain injury. Brain injury, 29(3), 374–379. https://doi.org/10.3109/02699052.2014.969312 (https://pubmed.ncbi.nlm.nih.gov/25356639/).
6. Vistisen, S. T., Hansen, T. K., Jensen, J., Nielsen, J. F., & Fleischer, J. (2014). Heart rate variability in neurorehabilitation patients with severe acquired brain injury. Brain injury, 28(2), 196–202. https://doi.org/10.3109/02699052.2013.860477 (https://pubmed.ncbi.nlm.nih.gov/24295072/).
7. Scheitz, J. F., Sposato, L. A., Schulz-Menger, J., Nolte, C. H., Backs, J., & Endres, M. (2022). Stroke-Heart Syndrome: Recent Advances and Challenges. Journal of the American Heart Association, 11(17), e026528. https://doi.org/10.1161/JAHA.122.026528 (https://pubmed.ncbi.nlm.nih.gov/36056731/).
8. Lee, Y., Walsh, R. J., Fong, M. W. M., Sykora, M., Doering, M. M., & Wong, A. W. K. (2021). Heart rate variability as a biomarker of functional outcomes in persons with acquired brain injury: Systematic review and meta-analysis. Neuroscience and biobehavioral reviews, 131, 737–754. https://doi.org/10.1016/j.neubiorev.2021.10.004 (https://pubmed.ncbi.nlm.nih.gov/34626686/).
9. Arakaki, X., Arechavala, R. J., Choy, E. H., Bautista, J., Bliss, B., Molloy, C., Wu, D. A., Shimojo, S., Jiang, Y., Kleinman, M. T., & Kloner, R. A. (2023). The connection between heart rate variability (HRV), neurological health, and cognition: A literature review. Frontiers in neuroscience, 17, 1055445. https://doi.org/10.3389/fnins.2023.1055445 (https://pubmed.ncbi.nlm.nih.gov/36937689/).
10. Agorastos, A., Mansueto, A. C., Hager, T., Pappi, E., Gardikioti, A., & Stiedl, O. (2023). Heart Rate Variability as a Translational Dynamic Biomarker of Altered Autonomic Function in Health and Psychiatric Disease. Biomedicines, 11(6), 1591. https://doi.org/10.3390/biomedicines11061591 (https://pubmed.ncbi.nlm.nih.gov/37371686/).
11. Buitrago-Ricaurte, N., Cintra, F., & Silva, G. S. (2020). Heart rate variability as an autonomic biomarker in ischemic stroke. Arquivos de neuro-psiquiatria, 78(11), 724–732. https://doi.org/10.1590/0004-282X20200087 (https://pubmed.ncbi.nlm.nih.gov/33331466/).
12. Dawson, J., Liu, C. Y., Francisco, G. E., Cramer, S. C., Wolf, S. L., Dixit, A., Alexander, J., Ali, R., Brown, B. L., Feng, W., DeMark, L., Hochberg, L. R., Kautz, S. A., Majid, A., O'Dell, M. W., Pierce, D., Prudente, C. N., Redgrave, J., Turner, D. L., Engineer, N. D., … Kimberley, T. J. (2021). Vagus nerve stimulation paired with rehabilitation for upper limb motor function after ischaemic stroke (VNS-REHAB): a randomised, blinded, pivotal, device trial. Lancet (London, England), 397(10284), 1545–1553. https://doi.org/10.1016/S0140-6736(21)00475-X (https://pubmed.ncbi.nlm.nih.gov/33894832/).
13. Lee, H., Lee, J. H., Hwang, M. H., & Kang, N. (2023). Repetitive transcranial magnetic stimulation improves cardiovascular autonomic nervous system control: A meta-analysis. Journal of affective disorders, 339, 443–453. https://doi.org/10.1016/j.jad.2023.07.039 (https://pubmed.ncbi.nlm.nih.gov/37459970/).
14. Mankoo, A., Roy, S., Davies, A., Panerai, R. B., Robinson, T. G., Brassard, P., Beishon, L. C., & Minhas, J. S. (2023). The role of the autonomic nervous system in cerebral blood flow regulation in stroke: A review. Autonomic neuroscience : basic & clinical, 246, 103082. https://doi.org/10.1016/j.autneu.2023.103082 (https://pubmed.ncbi.nlm.nih.gov/36870192/).
15. Matusik, P. S., Zhong, C., Matusik, P. T., Alomar, O., & Stein, P. K. (2023). Neuroimaging Studies of the Neural Correlates of Heart Rate Variability: A Systematic Review. Journal of clinical medicine, 12(3), 1016. https://doi.org/10.3390/jcm12031016 (https://pubmed.ncbi.nlm.nih.gov/36769662/).
16. Ross, S. N., & Ware, K. (2013). Hypothesizing the body's genius to trigger and self-organize its healing: 25 years using a standardized neurophysics therapy. Frontiers in physiology, 4, 334. https://doi.org/10.3389/fphys.2013.00334 (https://pubmed.ncbi.nlm.nih.gov/24312056/).
17. Orgianelis, I., Merkouris, E., Kitmeridou, S., Tsiptsios, D., Karatzetzou, S., Sousanidou, A., Gkantzios, A., Christidi, F., Polatidou, E., Beliani, A., Tsiakiri, A., Kokkotis, C., Iliopoulos, S., Anagnostopoulos, K., Aggelousis, N., & Vadikolias, K. (2023). Exploring the Utility of Autonomic Nervous System Evaluation for Stroke Prognosis. Neurology international, 15(2), 661–696. https://doi.org/10.3390/neurolint15020042 (https://pubmed.ncbi.nlm.nih.gov/37218981/).
18. Riganello, F., Larroque, S. K., Di Perri, C., Prada, V., Sannita, W. G., & Laureys, S. (2019). Measures of CNS-Autonomic Interaction and Responsiveness in Disorder of Consciousness. Frontiers in neuroscience, 13, 530. https://doi.org/10.3389/fnins.2019.00530 (https://pubmed.ncbi.nlm.nih.gov/31293365/).
19. Ruffle, J. K., Hyare, H., Howard, M. A., Farmer, A. D., Apkarian, A. V., Williams, S. C. R., Aziz, Q., & Nachev, P. (2021). The autonomic brain: Multi-dimensional generative hierarchical modelling of the autonomic connectome. Cortex; a journal devoted to the study of the nervous system and behavior, 143, 164–179. https://doi.org/10.1016/j.cortex.2021.06.012 (https://pubmed.ncbi.nlm.nih.gov/34438298/).
20. Siepmann, M., Weidner, K., Petrowski, K., & Siepmann, T. (2022). Heart Rate Variability: A Measure of Cardiovascular Health and Possible Therapeutic Target in Dysautonomic Mental and Neurological Disorders. Applied psychophysiology and biofeedback, 47(4), 273–287. https://doi.org/10.1007/s10484-022-09572-0 (https://pubmed.ncbi.nlm.nih.gov/36417141/).
En el episodio de hoy, hablo de un tema que se escapa un poco de la temática habitual del podcast. Este episodio es una “curiosidad de la neurociencia”; una indagación en un tema que desde hace muchos años me ha llamado la atención. Se trata de los savants. Los savants son personas que bien porque han nacido con problemas en el desarrollo o por una lesión cerebral adquirida, son capaces de tener habilidades extraordinarias. Parece ser que la mitad de los savant son autistas, uno de cada diez autistas es savant y uno de cada mil individuos que tienen dañado el cerebro o padecen retraso mental. Son personas que, sin necesidad de entrenamiento, aprendizaje o interés previo, tienen unas habilidades increíbles, sobre todo en el campo de la música, el cálculo o el dibujo.
Referencias del episodio:
1. Treffert D. A. (2014). Savant syndrome: realities, myths and misconceptions. Journal of autism and developmental disorders, 44(3), 564–571. (https://pubmed.ncbi.nlm.nih.gov/23918440/).
2. Treffert DA. The savant syndrome: an extraordinary condition. A synopsis: past, present, future. Philos Trans R Soc Lond B Biol Sci. 2009 May 27;364(1522):1351-7 (https://www.ncbi.nlm.nih.gov/pmc/articles/PMC2677584/).
3. Barr MW. Some notes on echolalia, with the report of an extraordinary case. J Nerv Ment Dis 1898;25:20-30 (https://zenodo.org/record/1734113).
4. Mishkin M, Malamut B, Bachevalier J. Memories and habits: two neural systems. In: Lynch G, McGaugh JL, Weinberger NM, editors. Neurobiology of learning and memory. New York: Guilford Press;1984. p.65-77.
5. Kapur N. (1996). Paradoxical functional facilitation in brain-behaviour research. A critical review. Brain : a journal of neurology, 119 ( Pt 5), 1775–1790.https://doi.org/10.1093/brain/119.5.1775 (https://pubmed.ncbi.nlm.nih.gov/9236635/).
6. Boso, M., Emanuele, E., Prestori, F., Politi, P., Barale, F., & D'Angelo, E. (2010). Autism and genius: is there a link? The involvement of central brain loops and hypotheses for functional testing. Functional neurology, 25(1), 15–20 (https://pubmed.ncbi.nlm.nih.gov/20630121/).
7. Snyder, A. W., Mulcahy, E., Taylor, J. L., Mitchell, D. J., Sachdev, P., & Gandevia, S. C. (2003). Savant-like skills exposed in normal people by suppressing the left fronto-temporal lobe. Journal of integrative neuroscience, 2(2), 149–158. https://doi.org/10.1142/s0219635203000287 (https://pubmed.ncbi.nlm.nih.gov/15011267/).
8. Snyder, A. W., & Thomas, M. (1997). Autistic artists give clues to cognition. Perception, 26(1), 93–96. https://doi.org/10.1068/p260093 (https://pubmed.ncbi.nlm.nih.gov/9196693/).
9. Humphrey, N. (1998). Cave Art, Autism, and the Evolution of the Human Mind. Cambridge Archaeological Journal, 8(2), 165-191. doi:10.1017/S0959774300001827 (https://www.cambridge.org/core/journals/cambridge-archaeological-journal/article/abs/cave-art-autism-and-the-evolution-of-the-human-mind/7E969D1ACAB536BD809348B9B4FE5C4D#).
10. Spikins, P., Scott, C. & Wright, B. (2018). How Do We Explain ‛Autistic Traits’ in European Upper Palaeolithic Art?. Open Archaeology, 4(1), 262-279. https://doi.org/10.1515/opar-2018-0016 (https://www.degruyter.com/document/doi/10.1515/opar-2018-0016/html#APA).
11. Folgerø, P. O., Johansson, C., & Stokkedal, L. H. (2021). The Superior Visual Perception Hypothesis: Neuroaesthetics of Cave Art. Behavioral sciences (Basel, Switzerland), 11(6), 81. https://doi.org/10.3390/bs11060081 (https://pubmed.ncbi.nlm.nih.gov/34073168/).
12. Lai G, Pantazatos SP, Schneider H, Hirsch J. Neural systems for speech and song in autism. Brain. 2012 Mar;135(Pt 3):961-75. doi: 10.1093/brain/awr335. Epub 2012 Feb 1. PMID: 22298195; PMCID: PMC3286324 (https://www.ncbi.nlm.nih.gov/pmc/articles/PMC3286324/).
13. Beate H. A memoir of the savant syndrome. Bright splinters of the mind: a personal story of research with autistics savant. London: Jessica Kingsley Publishers; 2001. p. 160 (https://www.proquest.com/docview/198983834).
14. Muñoz-Yunta JA , Ortiz T, Amo C, Fernández-Lucas A, Maestú F, Palau-Baduell M. El síndrome de savant o idiot savant. Rev Neurol 2003;36 (S1):157-0 (https://neurologia.com/articulo/2003061).
15. Geschwind, N., & Galaburda, A. M. (1985). Cerebral lateralization. Biological mechanisms, associations, and pathology: I. A hypothesis and a program for research. Archives of neurology, 42(5), 428–459. https://doi.org/10.1001/archneur.1985.04060050026008 (https://pubmed.ncbi.nlm.nih.gov/3994562/).
16. Navarro-Pardo E, Alonso-Esteban Y, Alcantud-Marin F, Murphy M. Do Savant Syndrome and Autism Spectrum Disorders Share Sex Differences? A Comprehensive Review. Soa Chongsonyon Chongsin Uihak. 2023 Apr 1;34(2):117-124. doi: 10.5765/jkacap.230008. PMID: 37035793; PMCID: PMC10080262 (https://www.ncbi.nlm.nih.gov/pmc/articles/PMC10080262/).
17. Pring, L., Ryder, N., Crane, L., & Hermelin, B. (2010). Local and global processing in savant artists with autism. Perception, 39(8), 1094–1103. https://doi.org/10.1068/p6674 (https://pubmed.ncbi.nlm.nih.gov/20942360/).
18. Mottron L, Dawson M, Soulières I. Enhanced perception in savant syndrome: patterns, structure and creativity. Philos Trans R Soc Lond B Biol Sci. 2009 May 27;364(1522):1385-91. doi: 10.1098/rstb.2008.0333. PMID: 19528021; PMCID: PMC2677591 (https://www.ncbi.nlm.nih.gov/pmc/articles/PMC2677591/).
19. Park HO. Autism Spectrum Disorder and Savant Syndrome: A Systematic Literature Review. Soa Chongsonyon Chongsin Uihak. 2023 Apr 1;34(2):76-92. doi: 10.5765/jkacap.230003. PMID: 37035789; PMCID: PMC10080257 (https://www.ncbi.nlm.nih.gov/pmc/articles/PMC10080257/).
20. Treffert, D. A., & Treffert, D. A. (2021). The Sudden Savant: A New Form of Extraordinary Abilities. WMJ : official publication of the State Medical Society of Wisconsin, 120(1), 69–73 (https://pubmed.ncbi.nlm.nih.gov/33974770/).
21. Documental “Mentes prodigiosas”. https://www.youtube.com/playlist?list=PL10391BEC6C746C6D
22. Daniel Tammet: sinestesia numérica. https://www.youtube.com/watch?v=HqBzez4WKuU&ab_channel=nuecesyneuronas
23. Documental sobre savant en Discovery: https://www.youtube.com/watch?v=BhZTM-aB0lw&t=2290s&ab_channel=PROYECTOTHERAPI
24. Accidental Genius | Darold Treffert | TEDxFondduLac : https://www.youtube.com/watch?v=Wxe1PkyJev8&ab_channel=TEDxTalks
En este episodio, reflexiono sobre el uso de las tecnologías avanzadas en neurorrehabilitación; reflexión que conviene hacer tanto si uno está familiarizado con ellas como si no. De vez en cuando hay que pararse a pensar, pensar dónde estamos, hacia dónde vamos y qué marcos de pensamiento y práctica tenemos debajo de la reluciente estética de la tecnología. La reflexión la realizo desde el punto de vista clínico, aunque existen otros enfoques, como el empresarial y de gestión. En cualquier caso, lo importante es tener un conocimiento y experiencia suficientes para discernir los fundamentos de la aplicación de tecnología en neurorrehabilitación.
Referencias del episodio:
1. José López Sánchez (2023). Tecnologías actuales aplicadas a la neurorrehabilitación. Innovación en neurorrehabilitación. Parque Científico Universidad Miguel Hernández (https://www.youtube.com/watch?v=lEUPm8WAvqs&ab_channel=ParqueCient%C3%ADficoUMH).
2. Putrino, D., & Krakauer, J. W. (2023). Neurotechnology's Prospects for Bringing About Meaningful Reductions in Neurological Impairment. Neurorehabilitation and neural repair, 37(6), 356–366. https://doi.org/10.1177/15459683221137341 (https://pubmed.ncbi.nlm.nih.gov/36384334/).
3. International Industry Society in Advanced Rehabilitation Technology (IISART) (https://iisart.org/).
En el episodio de hoy, hablamos del bonito mundo de la doble tarea, un área que ya de por sí tiene sus características en la sociedad en general y que en el campo de la rehabilitación es una categoría fundamental que tarde o temprano hay que abordar. El tema es extenso a más no poder y ha sido un poco complicado hacer una síntesis, ya que tenemos información de estudios tanto a nivel general de lo que implica la atención a dos tareas, críticas sociales (desde la sociología), estudios de neuropsicología y toda la parte de entrenamiento dual en terapia física y en diferentes patologías. Para esta ocasión, lo que me propongo es dar un marco cultural y sociológico inicial que creo que es importante para entender la globalidad del asunto; después voy a introducir conceptos fundamentales relacionados con la doble tarea y la idea después es transitando hacia estudios de correlatos neurales de la doble tarea y cómo podemos entrenar esa habilidad en neurorrehabilitación, sobre todo en la que concierne a la terapia física.
Referencias del episodio:
1. Leone, C., Feys, P., Moumdjian, L., D'Amico, E., Zappia, M., & Patti, F. (2017). Cognitive-motor dual-task interference: A systematic review of neural correlates. Neuroscience and biobehavioral reviews, 75, 348–360. https://doi.org/10.1016/j.neubiorev.2017.01.010 (https://pubmed.ncbi.nlm.nih.gov/28104413/).
2. Kuo, H. T., Yeh, N. C., Yang, Y. R., Hsu, W. C., Liao, Y. Y., & Wang, R. Y. (2022). Effects of different dual task training on dual task walking and responding brain activation in older adults with mild cognitive impairment. Scientific reports, 12(1), 8490. https://doi.org/10.1038/s41598-022-11489-x (https://pubmed.ncbi.nlm.nih.gov/35589771/).
3. Li, K. Z. H., Bherer, L., Mirelman, A., Maidan, I., & Hausdorff, J. M. (2018). Cognitive Involvement in Balance, Gait and Dual-Tasking in Aging: A Focused Review From a Neuroscience of Aging Perspective. Frontiers in neurology, 9, 913. https://doi.org/10.3389/fneur.2018.00913 (https://pubmed.ncbi.nlm.nih.gov/30425679/).
4. Mac-Auliffe D, Chatard B, Petton M, Croizé AC, Sipp F, Bontemps B, Gannerie A, Bertrand O, Rheims S, Kahane P, Lachaux JP. The Dual-Task Cost Is Due to Neural Interferences Disrupting the Optimal Spatio-Temporal Dynamics of the Competing Tasks. Front Behav Neurosci. 2021 Aug 19;15:640178. doi: 10.3389/fnbeh.2021.640178. PMID: 34489652; PMCID: PMC8416616 (https://www.ncbi.nlm.nih.gov/pmc/articles/PMC8416616/).
5. McPhee, A. M., Cheung, T. C. K., & Schmuckler, M. A. (2022). Dual-task interference as a function of varying motor and cognitive demands. Frontiers in psychology, 13, 952245. https://doi.org/10.3389/fpsyg.2022.952245 (https://pubmed.ncbi.nlm.nih.gov/36248521/).
6. Piqueres-Juan I, Tirapu-Ustárroz J, García-Sala M. Paradigmas de ejecución dual: aspectos conceptuales. Rev Neurol 2021;72 (10):357-367 (https://neurologia.com/articulo/2020200).
7. Plummer, P., Eskes, G., Wallace, S., Giuffrida, C., Fraas, M., Campbell, G., Clifton, K. L., Skidmore, E. R., & American Congress of Rehabilitation Medicine Stroke Networking Group Cognition Task Force (2013). Cognitive-motor interference during functional mobility after stroke: state of the science and implications for future research. Archives of physical medicine and rehabilitation, 94(12), 2565–2574.e6. https://doi.org/10.1016/j.apmr.2013.08.002 (https://pubmed.ncbi.nlm.nih.gov/23973751/).
8. St George, R. J., Jayakody, O., Healey, R., Breslin, M., Hinder, M. R., & Callisaya, M. L. (2022). Cognitive inhibition tasks interfere with dual-task walking and increase prefrontal cortical activity more than working memory tasks in young and older adults. Gait & posture, 95, 186–191. https://doi.org/10.1016/j.gaitpost.2022.04.021 (https://pubmed.ncbi.nlm.nih.gov/35525151/).
9. Strobach T. (2020). The dual-task practice advantage: Empirical evidence and cognitive mechanisms. Psychonomic bulletin & review, 27(1), 3–14. https://doi.org/10.3758/s13423-019-01619-4 (https://pubmed.ncbi.nlm.nih.gov/31152433/).
10. Watanabe, K., & Funahashi, S. (2014). Neural mechanisms of dual-task interference and cognitive capacity limitation in the prefrontal cortex. Nature neuroscience, 17(4), 601–611. https://doi.org/10.1038/nn.3667 (https://pubmed.ncbi.nlm.nih.gov/24584049/).
11. Ángel L. Martínez Nogueras (2020). Un repaso al paradigma de tarea dual desde la neuropsicología (1ª parte). (https://neurobase.wordpress.com/2020/03/20/un-repaso-al-paradigma-de-tarea-dual-desde-la-neuropsicologia-1a-parte/).
12. Johann Hari (2023). El valor de la atención. Por qué nos la robaron y cómo recuperarla (https://www.planetadelibros.com/libro-el-valor-de-la-atencion/365202).
13. McIsaac, T. L., Lamberg, E. M., & Muratori, L. M. (2015). Building a framework for a dual task taxonomy. BioMed research international, 2015, 591475. https://doi.org/10.1155/2015/591475 (https://pubmed.ncbi.nlm.nih.gov/25961027/).
14. Rémy, F., Wenderoth, N., Lipkens, K., & Swinnen, S. P. (2010). Dual-task interference during initial learning of a new motor task results from competition for the same brain areas. Neuropsychologia, 48(9), 2517–2527. https://doi.org/10.1016/j.neuropsychologia.2010.04.026 (https://pubmed.ncbi.nlm.nih.gov/20434467/).
15. D'Esposito, M., Detre, J. A., Alsop, D. C., Shin, R. K., Atlas, S., & Grossman, M. (1995). The neural basis of the central executive system of working memory. Nature, 378(6554), 279–281. https://doi.org/10.1038/378279a0 (https://pubmed.ncbi.nlm.nih.gov/7477346/9.
16. Just, M. A., Carpenter, P. A., Keller, T. A., Emery, L., Zajac, H., & Thulborn, K. R. (2001). Interdependence of nonoverlapping cortical systems in dual cognitive tasks. NeuroImage, 14(2), 417–426. https://doi.org/10.1006/nimg.2001.0826 (https://pubmed.ncbi.nlm.nih.gov/11467915/).
En este episodio, damos comienzo a uno de los proyectos más importantes y ambiciosos de Hemispherics. Nada menos que exponer lo fundamental de la neurofisiología aplicada a la rehabilitación del miembro superior tras una lesión neurológica.
Para este episodio, trataré de resumir el conocimiento respecto a la vía reticuloespinal y su relación con la corticoespinal y la recuperación motora y lo hilaré con el conocimiento de la sinergia flexora, los estudios con sistemas de soporte de peso y robóticos para el miembro superior. El objetivo es entender el por qué, el fundamento de los sistemas de soporte de peso y robóticos y qué puede estar ocurriendo en el cerebro para que se produzcan esos fenotipos de miembro superior.
Referencias del episodio:
1. Barker, R. N., Brauer, S., & Carson, R. (2009). Training-induced changes in the pattern of triceps to biceps activation during reaching tasks after chronic and severe stroke. Experimental brain research, 196(4), 483–496. https://doi.org/10.1007/s00221-009-1872-8 (https://pubmed.ncbi.nlm.nih.gov/19504088/).
2. Crocher, V., Fong, J., Bosch, T.J., Tan, Y., Mareels, I.M., & Oetomo, D. (2018). Upper Limb Deweighting Using Underactuated End-Effector-Based Backdrivable Manipulanda. IEEE Robotics and Automation Letters, 3, 2116-2122 (https://www.semanticscholar.org/paper/Upper-Limb-Deweighting-Using-Underactuated-Crocher-Fong/6624232dd6ca4e3bae776f684e5fb9e8acc0fc05).
3. Dewald, J. P., Pope, P. S., Given, J. D., Buchanan, T. S., & Rymer, W. Z. (1995). Abnormal muscle coactivation patterns during isometric torque generation at the elbow and shoulder in hemiparetic subjects. Brain : a journal of neurology, 118 ( Pt 2), 495–510. https://doi.org/10.1093/brain/118.2.495 (https://pubmed.ncbi.nlm.nih.gov/7735890/).
4. Dewald, J. P., Sheshadri, V., Dawson, M. L., & Beer, R. F. (2001). Upper-limb discoordination in hemiparetic stroke: implications for neurorehabilitation. Topics in stroke rehabilitation, 8(1), 1–12. https://doi.org/10.1310/WA7K-NGDF-NHKK-JAGD (https://pubmed.ncbi.nlm.nih.gov/14523747/).
5. Ellis, M. D., Carmona, C., Drogos, J., & Dewald, J. P. A. (2018). Progressive Abduction Loading Therapy with Horizontal-Plane Viscous Resistance Targeting Weakness and Flexion Synergy to Treat Upper Limb Function in Chronic Hemiparetic Stroke: A Randomized Clinical Trial. Frontiers in neurology, 9, 71. https://doi.org/10.3389/fneur.2018.00071 (https://pubmed.ncbi.nlm.nih.gov/29515514/).
6. Fong, J., Crocher, V., Haddara, R., Ackland, D., Galea, M., Tan, Y., & Oetomo, D. (2018). Effect Of Arm Deweighting Using End-Effector Based Robotic Devices On Muscle Activity. Annual International Conference of the IEEE Engineering in Medicine and Biology Society. IEEE Engineering in Medicine and Biology Society. Annual International Conference, 2018, 2470–2474. https://doi.org/10.1109/EMBC.2018.8512773 (https://pubmed.ncbi.nlm.nih.gov/30440908/).
7. Hammerbeck, U., Tyson, S. F., Samraj, P., Hollands, K., Krakauer, J. W., & Rothwell, J. (2021). The Strength of the Corticospinal Tract Not the Reticulospinal Tract Determines Upper-Limb Impairment Level and Capacity for Skill-Acquisition in the Sub-Acute Post-Stroke Period. Neurorehabilitation and neural repair, 35(9), 812–822. https://doi.org/10.1177/15459683211028243 (https://pubmed.ncbi.nlm.nih.gov/34219510/).
8. Kopke, J. V., Hargrove, L. J., & Ellis, M. D. (2021). Coupling of shoulder joint torques in individuals with chronic stroke mirrors controls, with additional non-load-dependent negative effects in a combined-torque task. Journal of neuroengineering and rehabilitation, 18(1), 134. https://doi.org/10.1186/s12984-021-00924-1 (https://pubmed.ncbi.nlm.nih.gov/34496876/).
9. McPherson, J. G., Chen, A., Ellis, M. D., Yao, J., Heckman, C. J., & Dewald, J. P. A. (2018). Progressive recruitment of contralesional cortico-reticulospinal pathways drives motor impairment post stroke. The Journal of physiology, 596(7), 1211–1225. https://doi.org/10.1113/JP274968 (https://pubmed.ncbi.nlm.nih.gov/29457651/).
10. McPherson, L. M., & Dewald, J. P. A. (2022). Abnormal synergies and associated reactions post-hemiparetic stroke reflect muscle activation patterns of brainstem motor pathways. Frontiers in neurology, 13, 934670. https://doi.org/10.3389/fneur.2022.934670 (https://pubmed.ncbi.nlm.nih.gov/36299276/).
11. Miller, L. C., Ruiz-Torres, R., Stienen, A. H., & Dewald, J. P. (2009). A wrist and finger force sensor module for use during movements of the upper limb in chronic hemiparetic stroke. IEEE transactions on bio-medical engineering, 56(9), 2312–2317. https://doi.org/10.1109/TBME.2009.2026057 (https://pubmed.ncbi.nlm.nih.gov/19567336/).
12. Miller, L. C., & Dewald, J. P. (2012). Involuntary paretic wrist/finger flexion forces and EMG increase with shoulder abduction load in individuals with chronic stroke. Clinical neurophysiology : official journal of the International Federation of Clinical Neurophysiology, 123(6), 1216–1225. https://doi.org/10.1016/j.clinph.2012.01.009 (https://pubmed.ncbi.nlm.nih.gov/22364723/).
13. Prange, G. B., Jannink, M. J., Stienen, A. H., van der Kooij, H., Ijzerman, M. J., & Hermens, H. J. (2009). Influence of gravity compensation on muscle activation patterns during different temporal phases of arm movements of stroke patients. Neurorehabilitation and neural repair, 23(5), 478–485. https://doi.org/10.1177/1545968308328720 (https://pubmed.ncbi.nlm.nih.gov/19190089/).
14. Runnalls, K. D., Anson, G., & Byblow, W. D. (2015). Partial weight support of the arm affects corticomotor selectivity of biceps brachii. Journal of neuroengineering and rehabilitation, 12, 94. https://doi.org/10.1186/s12984-015-0085-6 (https://pubmed.ncbi.nlm.nih.gov/26502933/).
15. Runnalls, K. D., Anson, G., & Byblow, W. D. (2017). Posture interacts with arm weight support to modulate corticomotor excitability to the upper limb. Experimental brain research, 235(1), 97–107. https://doi.org/10.1007/s00221-016-4775-5 (https://pubmed.ncbi.nlm.nih.gov/27639400/).
16. Runnalls, K. D., Ortega-Auriol, P., McMorland, A. J. C., Anson, G., & Byblow, W. D. (2019). Effects of arm weight support on neuromuscular activation during reaching in chronic stroke patients. Experimental brain research, 237(12), 3391–3408. https://doi.org/10.1007/s00221-019-05687-9 (https://pubmed.ncbi.nlm.nih.gov/31728596/).
17. Runnalls, K. D., Anson, G., Wolf, S. L., & Byblow, W. D. (2014). Partial weight support differentially affects corticomotor excitability across muscles of the upper limb. Physiological reports, 2(12), e12183. https://doi.org/10.14814/phy2.12183 (https://pubmed.ncbi.nlm.nih.gov/25501435/).
18. Sukal, T. M., Ellis, M. D., & Dewald, J. P. (2007). Shoulder abduction-induced reductions in reaching work area following hemiparetic stroke: neuroscientific implications. Experimental brain research, 183(2), 215–223. https://doi.org/10.1007/s00221-007-1029-6 (https://pubmed.ncbi.nlm.nih.gov/17634933/).
19. Wu, W., Fong, J., Crocher, V., Lee, P. V. S., Oetomo, D., Tan, Y., & Ackland, D. C. (2018). Modulation of shoulder muscle and joint function using a powered upper-limb exoskeleton. Journal of biomechanics, 72, 7–16. https://doi.org/10.1016/j.jbiomech.2018.02.019 (https://pubmed.ncbi.nlm.nih.gov/29506759/).
20. Dewald, J.P.A., Ellis, M.D., Acosta, A.M., Sohn, M.H., Plaisier, T.A.M. (2022). Implementation of Impairment-Based Neurorehabilitation Devices and Technologies Following Brain Injury. In: Reinkensmeyer, D.J., Marchal-Crespo, L., Dietz, V. (eds) Neurorehabilitation Technology. Springer, Cham. https://doi.org/10.1007/978-3-031-08995-4_5 (https://link.springer.com/chapter/10.1007/978-3-031-08995-4_5#citeas).
En este episodio, entrevisto a Sara Magallares Sánchez, logopeda especializada en neurorrehabilitación, formada en ciencia vocal y neurociencia cognitiva a nivel de Máster, con formación además transversal en neurorrehabilitación, como estimulación basal, electroestimulación, entre otras formaciones. Ha trabajado en distintos hospitales y clínicas y actualmente trabaja en CEN - Centro Europeo de Neurociencias, dentro de un marco de terapias intensivas.
Hablamos de logopedia y, en concreto, de la disartria, desde los conceptos básicos asociados, sus tipos hasta su evaluación y tratamiento.
Referencias del episodio:
Libros:
- Duffy, J. R. (2019). Motor Speech Disorders: Substrates, Differential Diagnosis, and Management (4th ed.). Elsevier.
- Schick, T. (2022). Functional Electrical Stimulation in Neurorehabilitation: Synergy Effects of Technology and Therapy. 1st Edition. Springer. https://doi.org/10.1007/978-3-030-90123-3
Artículos:
- Summaka, M., Hannoun, S., Harati, H. et al. (2022). Neuroanatomical Regions Associated With Non-progressive Dysarthria Post-Stroke: A Systematic Review. BMC Neurol 22, 353. https://doi.org/10.1186/s12883-022-02877-x
- Page, A.D.; Yorkston, K.M. (2022). Communicative Participation in Dysarthria: Perspectives for Management. Brain Sci 12, 420. https://doi.org/10.3390/brainsci12040420
- Borrie, S.A.; Wynn, C.J.; Berisha, V.; Barrett, T.S. (2022) From Speech Acoustics to Communicative Participation in Dysarthria: Toward a Causal Framework. J. Speech Hear. Disord. 65, 405–418.
Vídeos:
- https://www.youtube.com/watch?v=lCyRuhssJLc
En el episodio de hoy, os traigo el resumen de un artículo muy interesante y completo sobre la bradicinesia y los conceptos relacionados; es una revisión muy completa sobre cómo podemos comprender actualmente la bradicinesia como desorden de red y todo lo que la circunscribe a nivel clínico y neuropatológico. Lo que hacen en la revisión primero es ver los problemas de la terminología y la caracterización de la bradicinesia en estudios clínicos y experimentales en el Parkinson. Después se enfocan en la fisiopatología de la bradicinesia discutiendo el papel de los ganglios basales y la posible participación de otras estructuras, con la idea de aportar un modelo de red.
Referencias del episodio:
1. Bologna, M., Paparella, G., Fasano, A., Hallett, M., & Berardelli, A. (2020). Evolving concepts on bradykinesia. Brain : a journal of neurology, 143(3), 727–750. https://doi.org/10.1093/brain/awz344 (https://pubmed.ncbi.nlm.nih.gov/31834375/).
2. Postuma, R. B., Berg, D., Stern, M., Poewe, W., Olanow, C. W., Oertel, W., Obeso, J., Marek, K., Litvan, I., Lang, A. E., Halliday, G., Goetz, C. G., Gasser, T., Dubois, B., Chan, P., Bloem, B. R., Adler, C. H., & Deuschl, G. (2015). MDS clinical diagnostic criteria for Parkinson's disease. Movement disorders : official journal of the Movement Disorder Society, 30(12), 1591–1601. https://doi.org/10.1002/mds.26424 (https://pubmed.ncbi.nlm.nih.gov/26474316/).
3. Berg, D., Adler, C. H., Bloem, B. R., Chan, P., Gasser, T., Goetz, C. G., Halliday, G., Lang, A. E., Lewis, S., Li, Y., Liepelt-Scarfone, I., Litvan, I., Marek, K., Maetzler, C., Mi, T., Obeso, J., Oertel, W., Olanow, C. W., Poewe, W., Rios-Romenets, S., … Postuma, R. B. (2018). Movement disorder society criteria for clinically established early Parkinson's disease. Movement disorders : official journal of the Movement Disorder Society, 33(10), 1643–1646. https://doi.org/10.1002/mds.27431 (https://pubmed.ncbi.nlm.nih.gov/30145841/).
4. Tinaz, S., Pillai, A. S., & Hallett, M. (2016). Sequence Effect in Parkinson's Disease Is Related to Motor Energetic Cost. Frontiers in neurology, 7, 83. https://doi.org/10.3389/fneur.2016.00083 (https://pubmed.ncbi.nlm.nih.gov/27252678/).
5. Bar-Gad, I., & Bergman, H. (2001). Stepping out of the box: information processing in the neural networks of the basal ganglia. Current opinion in neurobiology, 11(6), 689–695. https://doi.org/10.1016/s0959-4388(01)00270-7 (https://pubmed.ncbi.nlm.nih.gov/11741019/).
6. Lee, M. S., Lee, M. J., Conte, A., & Berardelli, A. (2018). Abnormal somatosensory temporal discrimination in Parkinson's disease: Pathophysiological correlates and role in motor control deficits. Clinical neurophysiology : official journal of the International Federation of Clinical Neurophysiology, 129(2), 442–447. https://doi.org/10.1016/j.clinph.2017.11.022 (https://pubmed.ncbi.nlm.nih.gov/29304419/).
7. Tinkhauser, G., Pogosyan, A., Tan, H., Herz, D. M., Kühn, A. A., & Brown, P. (2017). Beta burst dynamics in Parkinson's disease OFF and ON dopaminergic medication. Brain : a journal of neurology, 140(11), 2968–2981. https://doi.org/10.1093/brain/awx252 (https://pubmed.ncbi.nlm.nih.gov/29053865/).
En el episodio de hoy, os traigo un tema muy interesante, un tema del que nadie escapa; todos hemos estudiado esto en algún momento de nuestra carrera o de nuestra especialización en el campo de la neurología. Hay una serie de elementos de la neurociencia que todos conocemos, que son transversales, que son muchas veces el punto de partida para explicar grandes dominios de la neurología. Y así es este caso, el caso del famoso homúnculo de Penfield; 'Homúnculo' o 'pequeño hombre', que tiene toda una historia detrás y por supuesto numerosos desarrollos científicos posteriores que han enriquecido y siguen enriqueciendo nuestra comprensión del sistema nervioso. He dividido este episodio en tres partes: 1) Historia del homúnculo; 2) Críticas al homúnculo; 3) Del homúnculo a la Interfaz Mente-Cuerpo.
Referencias del episodio:
1. Boldrey EB. The architectonic subdivision of the mammalian cerebral cortex : including a report of electrical stimulation of one hundred and five human cerebral cortices. Canada: McGill University; 1936 (https://www.proquest.com/openview/136252d5427e84161016fcbea2689e24/1?pq-origsite=gscholar&cbl=18750&diss=y).
2. Penfield W, Boldrey EB. Somatic motor and sensory representation in the cerebral cortex of man as studied by electrical stimulation. Brain. 1937;60: 389-443 (https://academic.oup.com/brain/article-abstract/60/4/389/332082?redirectedFrom=fulltext).
3. Penfield W, Rasmussen T. The Cerebral Cortex of Man. New York, NY: Macmillan; 1950 (https://psycnet.apa.org/record/1951-01483-000).
4. Tamè L et al. Bilateral representations of touch in the primary somatosensory cortex. Cogn Neuropsychol. 2016 Feb-Mar;33(1-2):48-66 (https://pubmed.ncbi.nlm.nih.gov/27314449/).
5. Sallés L, Gironès X, Lafuente JV. Organización motora del córtex cerebral y el papel del sistema de las neuronas espejo. Repercusiones clínicas para la rehabilitación. Med Clin (Barc). 2015 Jan 6;144(1):30-4 (https://pubmed.ncbi.nlm.nih.gov/24613375/).
6. Saadon-Grosman N, Loewenstein Y, Arzy S. The 'creatures' of the human cortical somatosensory system. Brain Commun. 2020 Jan 17;2(1):fcaa003 (https://pubmed.ncbi.nlm.nih.gov/32954277/).
7. Ravits J, Stack J. The lower motor neuron homunculus. Brain. 2022 Nov 21;145(11):3727-3729 (https://pubmed.ncbi.nlm.nih.gov/36029046/).
8. Parpia P. Reappraisal of the somatosensory homunculus and its discontinuities. Neural Comput. 2011 Dec;23(12):3001-15 (https://pubmed.ncbi.nlm.nih.gov/21732862/).
9. Morishita T, Miki K, Inoue T. Penfield Homunculus and Recent Advances in Brain Mapping. World Neurosurg. 2020 Feb;134:515-517 (https://pubmed.ncbi.nlm.nih.gov/31785433/).
10. Gandhoke GS et al. Edwin Boldrey and Wilder Penfield's Homunculus: A Life Given by Mrs. Cantlie (In and Out of Realism). World Neurosurg. 2019 Dec;132:377-388 (https://pubmed.ncbi.nlm.nih.gov/31470165/).
11. Iorio-Morin C, Mathieu D. Perspective on the Homunculus, the History of Cerebral Localization, and Evolving Modes of Data Representation. World Neurosurg. 2020 Mar;135:42-47 (https://pubmed.ncbi.nlm.nih.gov/31778834/).
12. Evan Gordon et al. A mind-body interface alternates with effector-specific regions in motor cortex. bioRxiv 2022.10.26.513940 (https://www.biorxiv.org/content/10.1101/2022.10.26.513940v1).
13. Neuroccino 7th Nov - A mind-body interface alternates with effector-specific regions in motor cortex (https://www.youtube.com/watch?v=vd-zoWdcOgI&ab_channel=ClinicalNeuroanatomySeminars).
14. Di Noto PM et al. The hermunculus: what is known about the representation of the female body in the brain? Cerebral Cortex (New York, N.Y. : 1991). 2013 May;23(5):1005-1013 (https://europepmc.org/article/med/22510528).
15. Haven Wright, Preston Foerder; The Missing Female Homunculus. Leonardo 2021; 54 (6): 653–656 (https://direct.mit.edu/leon/article-abstract/54/6/653/97296/The-Missing-Female Homunculus?redirectedFrom=fulltext).
16. Los homúnculos de Penfield – Blog Dr. José Ramón Alonso (https://jralonso.es/2014/04/26/los-homunculos-de-penfield/).
En el episodio de hoy, hablo del síndrome del empujador o patrón empujador, característico de algunos pacientes que han sufrido un ictus e incluso otras lesiones cerebrales. También llamado pusher, lateropulsión o empuje contraversivo, es un fenómeno neurológico que caracteriza la postura y el movimiento de algunos pacientes. En el episodio explico las generalidades, neuroanatomía, valoración y estrategias de tratamiento.
Referencias del episodio:
1. Karnath HO, Broetz D. Understanding and treating "pusher syndrome". Phys Ther. 2003 (https://pubmed.ncbi.nlm.nih.gov/14640870/).
2. Karnath HO, Johannsen L, Broetz D, Küker W. Posterior thalamic hemorrhage induces "pusher syndrome". Neurology. 2005 (https://pubmed.ncbi.nlm.nih.gov/15781819/).
3. Roller, Margaret L. DPT, MS. The ‘Pusher Syndrome’. Journal of Neurologic Physical Therapy: 2004 (https://journals.lww.com/jnpt/Fulltext/2004/03000/The__Pusher_Syndrome_.4.aspx).
4. Pérennou DA et al. Lateropulsion, pushing and verticality perception in hemisphere stroke: a causal relationship? Brain. 2008 (https://pubmed.ncbi.nlm.nih.gov/18678565/).
5. Bergmann J et al. The Subjective Postural Vertical Determined in Patients with Pusher Behavior During Standing. Top Stroke Rehabil. 2016 (https://pubmed.ncbi.nlm.nih.gov/27077977/).
6. Dai S et al. Balance, Lateropulsion, and Gait Disorders in Subacute Stroke. Neurology. 2021 (https://pubmed.ncbi.nlm.nih.gov/33177223/).
7. Nolan J, Godecke E, Singer B. The association between contraversive lateropulsion and outcomes post stroke: A systematic review. Top Stroke Rehabil. 2022 (https://pubmed.ncbi.nlm.nih.gov/33648434/).
8. Karnath HO, Ferber S, Dichgans J. The origin of contraversive pushing: evidence for a second graviceptive system in humans. Neurology. 2000 (https://pubmed.ncbi.nlm.nih.gov/11087771/).
9. Babyar SR et al. Lesion Localization of Poststroke Lateropulsion. Stroke. 2019 (https://pubmed.ncbi.nlm.nih.gov/31009350/).
10. Lee KB et al. Is Lateropulsion Really Related with a Specific Lesion of the Brain? Brain Sci. 2021 (https://pubmed.ncbi.nlm.nih.gov/33802116/).
11. Rosenzopt et al. Thalamocortical networks involved in Pusher Syndrome. bioRxiv. 2022 (https://www.biorxiv.org/content/10.1101/2022.10.12.511887v1).
12. Babyar SR et al. Clinical examination tools for lateropulsion or pusher syndrome following stroke: a systematic review of the literature. Clin Rehabil. 2009 (https://pubmed.ncbi.nlm.nih.gov/19403555/).
13. Bergmann J et al. A new cutoff score for the Burke Lateropulsion Scale improves validity in the classification of pusher behavior in subactue stroke patients. Gait Posture. 2019 (https://pubmed.ncbi.nlm.nih.gov/30623845/).
14. Martín Nieto, Ana (2018) Traducción, adaptación cultural y validación al castellano de las escalas "Scale for contraversive pushing" y "Burke laterospulsion scale". [Tesis] (https://eprints.ucm.es/id/eprint/46327/).
15. Paci M et al. Treatment approaches for pusher behaviour: a scoping review. Top Stroke Rehabil. 2022 (https://pubmed.ncbi.nlm.nih.gov/35156566/).
16. Bergmann J et al. Robot-assisted gait training to reduce pusher behavior: A randomized controlled trial. Neurology. 2018 (https://pubmed.ncbi.nlm.nih.gov/30171076/).
17. Romick-Sheldon D, Kimalat A. Novel Treatment Approach to Contraversive Pushing after Acute Stroke: A Case Report. Physiother Can. 2017 (https://pubmed.ncbi.nlm.nih.gov/30369698/).
18. Pardo V, Galen S. Treatment interventions for pusher syndrome: A case series. NeuroRehabilitation. 2019 (https://pubmed.ncbi.nlm.nih.gov/30814367/).
En esta actualización sobre espasticidad, hablamos de dos estudios del 2022 que transmiten información interesante para los clínicos. El primer estudio es de Fujimura y colaboradores, quienes hablan de la relación entre la velocidad de movimiento pasivo y las respuestas cinemáticas en pacientes con espasticidad leve. Aportan información sobre cómo realizar la valoración manual del tono. El segundo estudio es el de Frenkel-Toledo y colaboradores y trata sobre un análisis de mapeado por vóxeles del cerebro para disociar la hemiparesia y la espasticidad a nivel de topografía lesional. Un estudio francamente innovador. Empiezan a verse estudios que sí que tienen en cuenta literatura reciente y crítica con el constructo de la espasticidad como entidad suprema.
Referencias del episodio:
(1) Fujimura K, Mukaino M, Itoh S, Miwa H, Itoh R, Narukawa D, Tanikawa H, Kanada Y, Saitoh E, Otaka Y. Requirements for Eliciting a Spastic Response With Passive Joint Movements and the Influence of Velocity on Response Patterns: An Experimental Study of Velocity-Response Relationships in Mild Spasticity With Repeated-Measures Analysis. Front Neurol. 2022 Mar 30;13:854125. doi: 10.3389/fneur.2022.854125. PMID: 35432169; PMCID: PMC9007406 (https://pubmed.ncbi.nlm.nih.gov/35432169/).
(2) Frenkel-Toledo S, Levin MF, Berman S, Liebermann DG, Baniña MC, Solomon JM, Ofir-Geva S, Soroker N. Shared and distinct voxel-based lesion-symptom mappings for spasticity and impaired movement in the hemiparetic upper limb. Sci Rep. 2022 Jun 17;12(1):10169. doi: 10.1038/s41598-022-14359-8. PMID: 35715476; PMCID: PMC9206020 (https://pubmed.ncbi.nlm.nih.gov/35715476/).
En episodios anteriores sobre los desórdenes de conciencia, hablábamos de la conciencia desde la filosofía y la neurociencia. También en otro episodio, introdujimos los diferentes desórdenes de conciencia, sus características y diferencias fundamentales. Sin lugar a dudas, la clave dentro de la valoración de estos desórdenes de conciencia está en la capacidad de dar un pronóstico de recuperación y, embebido en ello, la capacidad de detectar cambios de transición entre la vigilia sin respuesta y el estado de mínima conciencia.
Es importante conocer ciertas escalas y pruebas que se realizan para valorar distintos aspectos que están relacionados con la conciencia y con la transición entre, por ejemplo, una vigilia sin respuesta a una mínima conciencia. Podemos distinguir dos tipos de valoraciones: las valoraciones clínicas, que se pueden realizar a pie de cama, que básicamente sistematizan las respuestas a distintos estímulos o respuestas espontáneas; y por otro lado las valoraciones neurofisiológicas que tratan de valorar el sustrato de la conciencia que permite comprender las respuestas comportamentales de los pacientes.
Referencias del episodio:
1. Cuadernos FEDACE sobre daño cerebral adquirido: síndrome de vigilia sin respuesta y de mínima conciencia (2011) (https://fedace.org/files/MSCFEDACE/2016-10/17-19-28-40.admin.13_vigilia_conciencia.pdf).
2. Giacino JT, Kalmar K, Whyte J. The JFK Coma Recovery Scale-Revised: measurement characteristics and diagnostic utility. Arch Phys Med Rehabil. 2004 (https://pubmed.ncbi.nlm.nih.gov/15605342/).
3. Manual de la CRS-R (Coma Recovery Scale-Revised) (https://www.tbims.org/combi/crs/CRS%20Syllabus.pdf).
4. Noé E, Olaya J, Navarro MD, Noguera P, Colomer C, García-Panach J, Rivero S, Moliner B, Ferri J. Behavioral recovery in disorders of consciousness: a prospective study with the Spanish version of the Coma Recovery Scale-Revised. Arch Phys Med Rehabil. 2012 (https://pubmed.ncbi.nlm.nih.gov/22277244/).
5. Schnakers C, Vanhaudenhuyse A, Giacino J, Ventura M, Boly M, Majerus S, Moonen G, Laureys S. Diagnostic accuracy of the vegetative and minimally conscious state: clinical consensus versus standardized neurobehavioral assessment. BMC Neurol. 2009 (https://pubmed.ncbi.nlm.nih.gov/19622138/).
6. Shiel A, Horn SA, Wilson BA, Watson MJ, Campbell MJ, McLellan DL. The Wessex Head Injury Matrix (WHIM) main scale: a preliminary report on a scale to assess and monitor patient recovery after severe head injury. Clin Rehabil. 2000 (https://pubmed.ncbi.nlm.nih.gov/10945425/).
7. Turner-Stokes L, Bassett P, Rose H, Ashford S, Thu A. Serial measurement of Wessex Head Injury Matrix in the diagnosis of patients in vegetative and minimally conscious states: a cohort analysis. BMJ Open. 2015 (https://pubmed.ncbi.nlm.nih.gov/25900459/).
8. Zasler ND, Formisano R, Aloisi M. Pain in Persons with Disorders of Consciousness. Brain Sci. 2022 (https://pubmed.ncbi.nlm.nih.gov/35326257/).
9. Rossi Sebastiano D, Varotto G, Sattin D, Franceschetti S. EEG Assessment in Patients With Disorders of Consciousness: Aims, Advantages, Limits, and Pitfalls. Front Neurol. 2021 (https://pubmed.ncbi.nlm.nih.gov/33868153/).
10. Pruvost-Robieux E, Marchi A, Martinelli I, Bouchereau E, Gavaret M. Evoked and Event-Related Potentials as Biomarkers of Consciousness State and Recovery. J Clin Neurophysiol. 2022 (https://pubmed.ncbi.nlm.nih.gov/34474424/).
11. Kondziella D y cols. European Academy of Neurology guideline on the diagnosis of coma and other disorders of consciousness. Eur J Neurol. 2020 (https://pubmed.ncbi.nlm.nih.gov/32090418/).
12. Formisano R, Contrada M, Aloisi M, Ferri G, Schiattone S, Iosa M, Buzzi MG. Nociception Coma Scale with personalized painful stimulation versus standard stimulus in non-communicative patients with disorders of consciousness. Neuropsychol Rehabil. 2020 (https://pubmed.ncbi.nlm.nih.gov/31088203/).
13. Formisano R, Aloisi M, Iosa M, Contrada M, Rizza F, Sattin D, Leonardi M, D'Ippolito M. A new tool to assess responsiveness in disorders of consciousness (DoC): a preliminary study on the Brief Post-Coma Scale (BPCS). Neurol Sci. 2018 (https://pubmed.ncbi.nlm.nih.gov/29948469/).
14. Cortese MD, Arcuri F, Nemirovsky IE, Lucca LF, Tonin P, Soddu A, Riganello F. Nociceptive Response Is a Possible Marker of Evolution in the Level of Consciousness in Unresponsive Wakefulness Syndrome Patients. Front Neurosci. 2021 (https://pubmed.ncbi.nlm.nih.gov/34975378/).
15. Chatelle C, Thibaut A, Bruno MA, Boly M, Bernard C, Hustinx R, Schnakers C, Laureys S. Nociception coma scale-revised scores correlate with metabolism in the anterior cingulate cortex. Neurorehabil Neural Repair. 2014 (https://pubmed.ncbi.nlm.nih.gov/24065132/).
16. Chatelle C, Thibaut A, Whyte J, De Val MD, Laureys S, Schnakers C. Pain issues in disorders of consciousness. Brain Inj. 2014 (https://pubmed.ncbi.nlm.nih.gov/25099024/).
17. Lin K, Wroten M. Ranchos Los Amigos. 2021 (https://pubmed.ncbi.nlm.nih.gov/28846341/).
18. American Congress of Rehabilitation Medicine, Brain Injury-Interdisciplinary Special Interest Group, Disorders of Consciousness Task Force, Seel RT y cols. Assessment scales for disorders of consciousness: evidence-based recommendations for clinical practice and research. Arch Phys Med Rehabil. 2010 (https://pubmed.ncbi.nlm.nih.gov/21112421/).
19. Disorder of Consciousness & Cognitive Recovery Following TBI Levels 1-10 with Dr. Alan Weintraub (Craig Hospital) (https://www.youtube.com/watch?v=ZWJUfSWYppM&t=2516s&ab_channel=CraigHospital).
En este episodio, introducimos la controversia con la neurogénesis en el humano adulto, ya que hay diferentes estudios que no obtienen los mismos resultados que los estudios que afirman que existe la neurogénesis en el hipocampo adulto. Aportamos contexto histórico y detalles neurobiológicos que permiten abordar esta controversia con mayor rigor que el habitual en la divulgación en neurociencia y neurorrehabilitación. Entrevistamos a Jon Arellano, investigador en la Universidad de Yale, para aportar una visión crítica y actualizada de la controversia de la neurogénesis.
Referencias del episodio:
1. Snyder (2018). Questioning human neurogenesis (https://www.nature.com/articles/d41586-018-02629-3).
2. The Snyder Lab. WTF! No neurogenesis in humans?? http://snyderlab.com/2018/03/07/wtf-no-neurogenesis-in-humans/
3. Sorrells SF et al. Human hippocampal neurogenesis drops sharply in children to undetectable levels in adults. Nature. 2018 Mar 15;555(7696):377-381. doi: 10.1038/nature25975. Epub 2018 Mar 7. PMID: 29513649; PMCID: PMC6179355 (https://pubmed.ncbi.nlm.nih.gov/29513649/).
4. Dennis CV et al. Human adult neurogenesis across the ages: An immunohistochemical study. Neuropathol Appl Neurobiol. 2016 Dec;42(7):621-638. doi: 10.1111/nan.12337. Epub 2016 Aug 28. PMID: 27424496; PMCID: PMC5125837 (https://pubmed.ncbi.nlm.nih.gov/27424496/).
5. Moreno-Jiménez EP, Terreros-Roncal J, Flor-García M, Rábano A, Llorens-Martín M. Evidences for Adult Hippocampal Neurogenesis in Humans. J Neurosci. 2021 Mar 24;41(12):2541-2553. doi: 10.1523/JNEUROSCI.0675-20.2020. PMID: 33762406; PMCID: PMC8018741 (https://pubmed.ncbi.nlm.nih.gov/33762406/).
6. Moreno-Jiménez EP, Flor-García M, Terreros-Roncal J, Rábano A, Cafini F, Pallas-Bazarra N, Ávila J, Llorens-Martín M. Adult hippocampal neurogenesis is abundant in neurologically healthy subjects and drops sharply in patients with Alzheimer's disease. Nat Med. 2019 Apr;25(4):554-560. doi: 10.1038/s41591-019-0375-9. Epub 2019 Mar 25. PMID: 30911133 (https://pubmed.ncbi.nlm.nih.gov/30911133/).
7. Eriksson PS, Perfilieva E, Björk-Eriksson T, Alborn AM, Nordborg C, Peterson DA, Gage FH. Neurogenesis in the adult human hippocampus. Nat Med. 1998 Nov;4(11):1313-7. doi: 10.1038/3305. PMID: 9809557 (https://pubmed.ncbi.nlm.nih.gov/9809557/).
8. Cipriani S, Ferrer I, Aronica E, Kovacs GG, Verney C, Nardelli J, Khung S, Delezoide AL, Milenkovic I, Rasika S, Manivet P, Benifla JL, Deriot N, Gressens P, Adle-Biassette H. Hippocampal Radial Glial Subtypes and Their Neurogenic Potential in Human Fetuses and Healthy and Alzheimer's Disease Adults. Cereb Cortex. 2018 Jul 1;28(7):2458-2478. doi: 10.1093/cercor/bhy096. PMID: 29722804 (https://pubmed.ncbi.nlm.nih.gov/29722804/9.
9. Kempermann G, Gage FH, Aigner L, Song H, Curtis MA, Thuret S, Kuhn HG, Jessberger S, Frankland PW, Cameron HA, Gould E, Hen R, Abrous DN, Toni N, Schinder AF, Zhao X, Lucassen PJ, Frisén J. Human Adult Neurogenesis: Evidence and Remaining Questions. Cell Stem Cell. 2018 Jul 5;23(1):25-30. doi: 10.1016/j.stem.2018.04.004. Epub 2018 Apr 19. PMID: 29681514; PMCID: PMC6035081 (https://pubmed.ncbi.nlm.nih.gov/29681514/).
10. Jon I Arellano, Brian Harding, Jean-Leon Thomas, Adult Human Hippocampus: No New Neurons in Sight, Cerebral Cortex, Volume 28, Issue 7, July 2018, Pages 2479–2481, https://doi.org/10.1093/cercor/bhy106.
11. Leal-Galicia P, Chávez-Hernández ME, Mata F, Mata-Luévanos J, Rodríguez-Serrano LM, Tapia-de-Jesús A, Buenrostro-Jáuregui MH. Adult Neurogenesis: A Story Ranging from Controversial New Neurogenic Areas and Human Adult Neurogenesis to Molecular Regulation. Int J Mol Sci. 2021 Oct 25;22(21):11489. doi: 10.3390/ijms222111489. PMID: 34768919; PMCID: PMC8584254 (https://pubmed.ncbi.nlm.nih.gov/34768919/).
12. Boldrini M, Fulmore CA, Tartt AN, Simeon LR, Pavlova I, Poposka V, Rosoklija GB, Stankov A, Arango V, Dwork AJ, Hen R, Mann JJ. Human Hippocampal Neurogenesis Persists throughout Aging. Cell Stem Cell. 2018 Apr 5;22(4):589-599.e5. doi: 10.1016/j.stem.2018.03.015. PMID: 29625071; PMCID: PMC5957089 (https://pubmed.ncbi.nlm.nih.gov/29625071/).
13. Nogueira AB, Hoshino HSR, Ortega NC, Dos Santos BGS, Teixeira MJ. Adult human neurogenesis: early studies clarify recent controversies and go further. Metab Brain Dis. 2022 Jan;37(1):153-172. doi: 10.1007/s11011-021-00864-8. Epub 2021 Nov 5. PMID: 34739659 (https://pubmed.ncbi.nlm.nih.gov/34739659/).
14. Bernier PJ, Vinet J, Cossette M, Parent A. Characterization of the subventricular zone of the adult human brain: evidence for the involvement of Bcl-2. Neurosci Res. 2000 May;37(1):67-78. doi: 10.1016/s0168-0102(00)00102-4. PMID: 10802345 (https://pubmed.ncbi.nlm.nih.gov/10802345/).
15. Franjic D, Skarica M, Ma S, Arellano JI, Tebbenkamp ATN, Choi J, Xu C, Li Q, Morozov YM, Andrijevic D, Vrselja Z, Spajic A, Santpere G, Li M, Zhang S, Liu Y, Spurrier J, Zhang L, Gudelj I, Rapan L, Takahashi H, Huttner A, Fan R, Strittmatter SM, Sousa AMM, Rakic P, Sestan N. Transcriptomic taxonomy and neurogenic trajectories of adult human, macaque, and pig hippocampal and entorhinal cells. Neuron. 2022 Feb 2;110(3):452-469.e14. doi: 10.1016/j.neuron.2021.10.036. Epub 2021 Nov 18. PMID: 34798047; PMCID: PMC8813897 (https://pubmed.ncbi.nlm.nih.gov/34798047/.
16. Alvarez-Buylla A, Cebrian-Silla A, Sorrells SF, Nascimento MA, Paredes MF, Garcia-Verdugo JM, Yang Z, Huang EJ. Comment on "Impact of neurodegenerative diseases on human adult hippocampal neurogenesis". Science. 2022 Apr 15;376(6590):eabn8861. doi: 10.1126/science.abn8861. Epub 2022 Apr 15. PMID: 35420933 (https://pubmed.ncbi.nlm.nih.gov/35420933/).
17. Bergmann O, Liebl J, Bernard S, Alkass K, Yeung MS, Steier P, Kutschera W, Johnson L, Landén M, Druid H, Spalding KL, Frisén J. The age of olfactory bulb neurons in humans. Neuron. 2012 May 24;74(4):634-9. doi: 10.1016/j.neuron.2012.03.030. PMID: 22632721 (https://pubmed.ncbi.nlm.nih.gov/22632721/).
En este episodio, entrevisto a Gonzalo Varas, él es kinesiólogo por la Universidad Andrés Bello. Magíster en Terapia Manual Ortopédica, UNAB. Magíster en Ciencias Biológicas, Mención Neurociencias de la Universidad de Chile. Doctor en Ciencias de la Rehabilitación por la Universidad de Illinois, Chicago (EEUU). Es miembro y ex director de la Asociación Chilena de Ciencias del Movimiento y miembro de la Asociación Latinoamericana de Rehabilitación Neurológica.
A nivel clínico, es kinesiólogo especializado la Neurorrehabilitación, principalmente a la rehabilitación de personas adultas con secuela de lesión medular y lesión encefálica.
A nivel de investigación, se ha enfocado en el control y aprendizaje motor en contextos de lesión al sistema nervioso, control del equilibrio y la marcha en personas mayores y con lesión del sistema nervioso; y quizás más concretamente también ha investigado mucho el equilibrio reactivo.
Actualmente es Profesor Asistente de la Escuela de Kinesiología de la Universidad Finis Terrae, Chile y académico investigador en el Laboratorio de Fisiología del ejercicio y metabolismo de la Facultad de Medicina de la Universidad Finis Terrae.
Redes sociales de Gonzalo:
-Instagram: gonzalovaras_pt
-Researchgate: https://www.researchgate.net/profile/Gonzalo-Varas-Diaz
Referencias del episodio:
(1) Tesis Doctoral Gonzalo Varas: Effect of Cognitive, Impairment-Oriented and Task-Specific Interventions on Balance and Locomotion Control (https://indigo.uic.edu/articles/thesis/Effect_of_Cognitive_Impairment-Oriented_and_Task-Specific_Interventions_onBalance_and_Locomotion_Control/15261993).
(2) Wang S, Varas-Diaz G, Bhatt T. Muscle synergy differences between voluntary and reactive backward stepping (https://pubmed.ncbi.nlm.nih.gov/34326376/).
(3) Dakin CJ, Bolton DAE. Forecast or Fall: Prediction's Importance to Postural Control. Front Neurol. 2018 (https://pubmed.ncbi.nlm.nih.gov/30425680/).
(4) Kannan L, Vora J, Varas-Diaz G, Bhatt T, Hughes S. Does Exercise-Based Conventional Training Improve Reactive Balance Control among People with Chronic Stroke? Brain Sci. 2020 (https://pubmed.ncbi.nlm.nih.gov/33374957/).
(5) Varas-Diaz G, Cordo P, Dusane S, Bhatt T. Effect of robotic-assisted ankle training on gait in stroke participants: A case series study. Physiother Theory Pract. 2021 (https://pubmed.ncbi.nlm.nih.gov/34424126/).
(6) Varas-Diaz G, Kannan L, Bhatt T. Effect of Mental Fatigue on Postural Sway in Healthy Older Adults and Stroke Populations. Brain Sci. 2020 (https://pubmed.ncbi.nlm.nih.gov/32575383/).
(7) Bhatt T, Wening JD, Pai YC. Influence of gait speed on stability: recovery from anterior slips and compensatory stepping. Gait Posture. 2005 (https://pubmed.ncbi.nlm.nih.gov/15639393/).
En este episodio, entrevisto a Diego Martín Laxague. Diego Martín es terapeuta ocupacional especializado en la rehabilitación de la mano y de la extremidad superior, con experiencia en lesiones neurológicas, traumáticas y ortopédicas. Además está formado en técnicas ortoprotésicas y en la movilización instrumental de tejidos blandos. Es miembro de AETEMA (Asociación Española de Terapeutas de Mano). También es co-director de Hand Center (Asturias) y además, se dedica a la docencia y las formaciones sobre ferulaje neumático y NRX-Strap.
Le he invitado al podcast para que nos cuente su experiencia con la rehabilitación del miembro superior neurológico y especialmente con la parte estructural. Diego es un profesional que da muchas muestras de su trabajo diario a través de las redes sociales y estoy seguro de que es inspiración para muchos terapeutas como yo que disfrutamos con su creatividad a la hora de plantear ejercicios.
Redes sociales de Diego:
-Instagram: @martinlaxague_to
Referencias del episodio: