Discover
Under the Microscope
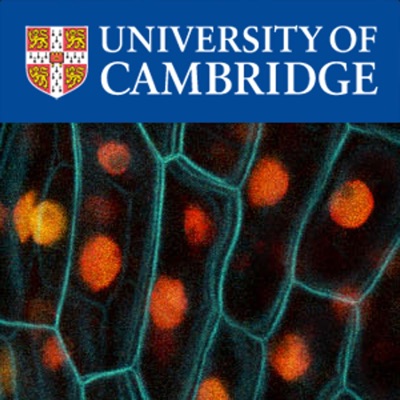
Under the Microscope
Author: Cambridge University
Subscribed: 58Played: 110Subscribe
Share
© 2013
Description
Cambridge University's Under the Microscope is a collection of videos that capture glimpses of the natural and man-made world in stunning close-up and convey the excitement of cutting-edge science in areas that range from beetle eyes to killer T-cells, from nano-wires to fish skeletons.
Logo image by Fernan Federici in the Haseloff Lab.
Logo image by Fernan Federici in the Haseloff Lab.
16 Episodes
Reverse
Matthew Kuo tells us how tiny worm faecal pellets affect how oil pipelines sit on the seabed.
Nanowires growing in real time. Each nanowire is roughly 400 atoms wide.
Dr Andrew Gillis explains how an elephant fish embryo lives off a large yellow yolk sack for 7 to 10 months before hatching out as a fish.
Dr Gillis:
“This is a picture of an elephant fish embryo. Elephant fish are cartilaginous fishes, and are distant cousins of sharks, skates and stingrays. The elephant fish lives in deep water off the coasts of Australia and New Zealand, but migrates annually into shallow coastal bays to lay their eggs. I study the embryonic development of elephant fish, by collecting their eggs by SCUBA diving at their egg-laying grounds. Normally, an elephant fish embryo will live in their egg and feed off of their yolk supply for 7 to 10 months before hatching out as a completely self-sufficient juvenile. However, these embryos may also be cultured outside of their egg cases, as seen here. This allows us to observe and photograph the development and growth of this unusual fish.”
The diameter of the petri dish in the elephant fish picture is 10cm.
More info:
http://www.pdn.cam.ac.uk/~jag93
Music by Peter Nickalls:
http://www.peternickalls.com
Many thanks:
Graduate School of Life Sciences
In this video Dr Sungjune Jung shows us the fluid structures produced by the impact of two liquid jets.
Dr Jung:
“This video shows the evolution of the flow structures generated from the collision of two liquid jets each with a radius of 420um. The jets were ejected from parallel cylindrical nozzles with an internal diameter of 0.85mm.
The collision of the jets resulted in various systems of behaviour which depend on the jet velocities and the liquid properties. We focus on the system where the impinging jets form a liquid sheet which then breaks up into a regular succession of ligaments and droplets, a so-called "fishbone" pattern. This high-speed imaging reveals a fish-like formation for the fluid:
the oval sheet with rims correspond to the fish head, the drops on thin ligaments to its body, and bigger free drops at the end to its tail. We are particularly interested in this fluid formation, because the fishbone phenomenon provides a simple and visual tool to evaluate the properties of inkjet printing fluids, with which the fishbone structure sensitively varies."
Many thanks to Prof Ian Hutchings, Dr Graham Martin and Dr Steve Hoath at Inkjet Research Centre, Department of Engineering.
More info:
Dr Jung's profile:
http://www.oe.phy.cam.ac.uk/people/oepdras/sjj37.htm
Inkjet Research Centre
http://www.ifm.eng.cam.ac.uk/pp/inkjet/
Department of Engineering
http://www.eng.cam.ac.uk/
Music by Intercontinental Music Lab
http://www.intercontinentalmusiclab.com
This is a beautiful image of human brain cells, which can now be grown from adult skin cells.
Yichen Shi:
"Brain neural stem cells derived from human skin cells: these stem cells express typical marker genes of brain neocortical stem cells, such as Pax6 (Red fluorescent labeled), and form a rosette structure resembling the transection of the neural tube."
The entire image is about 250 μm across (a really thick bit of human hair).
More info:
http://www.cam.ac.uk/research/news/brain-cells-created-from-patients-skin-cells
Picture taken by Yichen Shi in the Livesey Lab
http://www.gurdon.cam.ac.uk/~liveseylab/fjlhome/index.html
Voice over by Fred Lewsey.
Music by Peter Nickalls: http://www.peternickalls.com
PhD student Paola Cognigni shows us this beautiful image of a fruit fly’s brain and gut.
Paola Cognigni:
“This video shows the anatomical and functional connection between the brain and the gut in the fruit fly, Drosophila melanogaster. This work is carried out in Dr Irene Miguel-Aliaga's lab in the Department of Zoology as part of a research project that aims to find and explain the interactions between internal organs and their importance in growth and health.”
The brain is about 700 microns wide (the entire image is something like
1600 microns across): about the size of a pencil tip.
The image was taken in the Zoology Dept Imaging Facility on a Leica SP5 confocal system.
More info and images:
http://www.zoo.cam.ac.uk/zoostaff/miguel-aliaga/main.html
Music by Intercontinental Music Lab
http://www.intercontinentalmusiclab.com
Here we can see the underside of mouse tail skin.
Claire Cox:
"The epidermis, which is the outer layer of mammalian skin, is maintained by numerous stem cell populations. The identification of the factors involved in controlling these populations and thus epidermal maintenance is highly valuable. Not only will it provide information as to how a complex tissue is organised and controlled, the principles that are learnt can be applied to other tissues. Through the work that I am completing, I hope that I can also gain a perspective as to what goes wrong in disease processes such as skin cancer. Skin cancer is one of the most prevalent cancers in the world, and understanding what goes wrong and the factors involved could potentially lead to new ideas as to prevention and treatment."
The image is 700µm in width - this is about the size of the full stop in this sentence. About 5000 cells would fit on the surface of a full stop.
Many thanks to:
Dr Michaela Frye, Frye Lab members, Peter Humphreys, Margaret McLeish.
More info:
Wellcome Trust Centre For Stem Cell Research
http://www.cscr.cam.ac.uk
Department of Physiology Development and Neuroscience http://www.pdn.cam.ac.uk/
Claire Cox's profile:
http://www.cscr.cam.ac.uk/research/researchers-by-group/frye-lab/claire-cox
Graduate School of Life Sciences and its annual Poster and Image Competitions
http://www.biomed.cam.ac.uk/gradschool/comp/2011/index.html
Music by Peter Nickalls:
http://www.peternickalls.com
Find more Cambridge research here:
http://www.cam.ac.uk/research
Dr Andrew Gillis shows us an embryonic skate head and explains how the red denticles dotted all over it have very similar properties to human teeth.
Dr Gillis:
"This is a picture of the head of an embryonic skate (Leucoraja erinacea). A skate is a cartilaginous fish, closely related to sharks and stingrays. This embryo has been stained with dyes that colour the skeleton - cartilage is blue, and mineralised tissue is red - and then cleared with chemicals to make it transparent. The result is a specimen that shows the complex shape and arrangement of different skeletal tissues during embryonic development. I use this staining procedure to visualise the skeleton following experimental manipulation. This allows me to investigate how different genes and proteins are involved in controlling the formation and growth of different skeletal tissues in these fishes".
The skate image is approximately 3cm across.
More info:
http://www.pdn.cam.ac.uk/~jag93
Music by Peter Nickalls:
http://www.peternickalls.com
With thanks:
Graduate School of Life Sciences and its annual Poster and Image Competitions
http://www.biomed.cam.ac.uk/gradschool/comp/2011/index.html
Matt Benton shows us nuclei moving inside a beetle egg as a beetle embryo forms.
Matt Benton:
“For my PhD I am studying the embryonic development of the beetle, Tribolium castaneum. During development in this beetle, a large number cells must move together at a certain location of the egg to form the embryo proper. At the same time, other cells move to overlap the forming embryo, to protect it and help it grow. Currently, we only have a basic understanding of how these different groups of cells move. In my work I am trying to extend this understanding, and to learn how the movements of different groups of cells are controlled and coordinated. Together with the group of Michalis Averof, I am developing methods to allow the movements of these cells to be seen in live embryos. The beetle shown in this video has been genetically modified so that the nucleus of each cell is labelled with a fluorescent protein. By using a certain microscope, I am able to record the movements of these cells in 3D, as the embryo develops.
Many thanks to Michalis Averof for creating the nuclear-green fluorescent protein transgenic line shown in the movie, and to my PhD supervisor, Michael Akam, for supporting my work.”
The width of this egg is 300 micrometres, and the length is 600 micrometres (1 metre is 1,000,000 micrometres). So the width of this egg is roughly 3 times the width of a human hair.
The time span of the movie is about 5.5 hours.
Matt Benton’s profile:
http://www.zoo.cam.ac.uk/zoostaff/akam/benton.html
Music by Sophie Smith:
http://www.sophieasmith.co.uk
In this video Dr Ingrid Graz shows us a thin layer of gold on top of rubber. Cracks in the gold allow it to stretch and we can use this for stretchable electronics.
Dr Graz:
“Imagine a future mobile phone that can be wrapped around your wrist or an MP3 player that is integrated in your T-shirt. Stretchable electronics is a new evolution of electronics - the idea behind is to create electronic devices that can be rolled, flexed, deformed and even stretch like a rubber band. To enable stretchable electronics we use rubber such as silicone coated with a very thin layer of gold. The gold serves as stretchable conductor and can be elongated to twice its original length without electrical failure. The secret behind the stretchability lies within the microstructure. Tiny cracks in the film open up when it is stretched without damaging the film. This image shows a silicone rubber with a gold layer and an additional silicone layer to protect the electrode.”
The image is about 3x3mm.
Nanoscience Centre, University of Cambridge:
http://www.nanoscience.cam.ac.uk
Department of Engineering:
www.eng.cam.ac.uk
Music by Peter Nickalls:
http://www.peternickalls.com
In this video we see a killer T cell of the immune system attacking a cancer cell.
Professor Gillian Griffiths:
“Cells of the immune system protect the body against pathogens. If cells in our bodies are infected by viruses, or become cancerous, then killer cells of the immune system identify and destroy the affected cells. Cytotoxic T cells are very precise and efficient killers. They are able to destroy infected or cancerous cells, without destroying healthy cells surrounding them. The Wellcome Trust funded laboratory of Professor Gillian Griffiths, at the Cambridge Institute for Medical Research, investigates just how this is accomplished. By understanding how this works, we can develop ways to control killer cells. This will allow us to find ways to improve cancer therapies, and ameliorate autoimmune diseases caused when killer cells run amok and attack healthy cells in our bodies.”
Cytotoxic T cells are just 10 microns in length: approximately one-tenth the width of a human hair. These movies are 92 times real time.
The original footage shown was made by Alex Ritter, a PhD student on the NIH-OxCam programme, in the laboratory of Professor Gillian Griffiths at the Cambridge Institute for Medical Research and the Department of Medicine of the Clinical School of the University of Cambridge. The images were acquired using an Andor Revolution spinning disk system with an Olympus microscope. Professor Griffiths is a Wellcome Trust Principal Research Fellow.
Links for more information:
http://www.cimr.cam.ac.uk
http://www.immunology.cam.ac.uk/about
Music by Intercontinental Music Lab
http://www.intercontinentalmusiclab.com
In this video Dr Beverley Glover explains how a daisy is a collection of tiny flowers grouped together to make it look like a single big flower.
Dr Glover:
“The flowering plants (Angiosperms) form the dominant vegetation over most of the Earth’s land surface. They are found in all habitats except the Antarctic, and can tolerate an extraordinarily wide range of environmental conditions. All major human food crops are Angiosperms. We are interested in the evolution and development of the flower, one of the defining features of Angiosperms. The evolution of flowers changed the way in which plants reproduced, allowing them to use animals to carry their pollen around. Our research is particularly focussed on understanding how the features that make flowers attractive to insects evolved, and what the genetic control of their development is. We hope to be able to use this knowledge to improve pollination and yield of important crop plants and to help protect the great diversity of flowers and insect pollinators in the wild.
This image of a developing daisy flower head is part of our work on understanding different ways of attracting pollinators. All the plants in the daisy family use the same trick – by clustering together many tiny flowers they produce a structure that looks just like a single big flower. The daisies that grow in our lawns contain two different types of flowers – central radially symmetrical yellow ones, and an outer ring of bilaterally symmetrical white ones with a massively elongated petal structure. In this Scanning Electron Micrograph you can see the central yellow flowers at a very early stage of development, with the petals still folded over the centre of each little flower.”
Each of the round structures that will go on to be an individual flower is about 200 micrometres in diameter, or 1/5 of a millimetre. The whole image is about 1mm across.
Dr Glover’s profile:
http://www.plantsci.cam.ac.uk/research/beverleyglover.html
Department of Plant Sciences:
http://www.plantsci.cam.ac.uk
Music by Peter Nickalls:
http://www.peternickalls.com
Dr Tim Wilkinson is combining liquid crystals with nanotechnology to try and create 3D displays which would look like real life.
Dr Wilkinson: “Liquid crystal displays are now a commonplace technology from mobile phone displays to wide screen televisions. They are, however, still limited by the shape, size and speed of their pixels when they are used to display video images. This video shows microscope sequences of a new nanotechnology based liquid crystal pixel structure that will allow much higher resolution displays and even true 3D holographic displays to be fabricated in the future.”
The videos are all in real time. The scale varies from video to video, but the little dots which form a grid in most of them are all 10 μm apart (10th of diameter of a hair).
More info:
http://www-g.eng.cam.ac.uk/CMMPE/displays2d.html
Department of Engineering:
www.eng.cam.ac.uk
Music by Intercontinental Music Lab
http://www.intercontinentalmusiclab.com
In this video, we see a mouse embryo developing. Erica Watson tells us that studying this process helps us better understand human pregnancy.
Erica Watson:
“The development of a fetus is elegant yet complex. Amazingly, most fetuses undergo a highly orchestrated sequence of events during development to produce a healthy baby. This suggests that a baby can adapt to changes in the womb, such as to the availability of nutrients from its mother. But how do these environmental changes affect the baby’s health in later life? And is it possible that these adverse changes will alter the development of generations to follow? In other words, does the environment that a baby develops in affect its grandchildren’s growth and development? Our research aims to understand these questions using a mouse model with a genetic mutation that prevents the normal breakdown folic acid (a vitamin). This mutation alters the metabolism of a mouse and causes long-lasting effects on the generations to come. Our hope is to find out how environmental changes caused by a genetic mutation are perpetuated into subsequent generations, even when these generations do not carry the mutation.
Since humans and mice use similar genes during development, we can get valuable information from a mouse model about how an embryo and its placenta develop over time. Compared to a human pregnancy that lasts nine months, a mouse fetus develops quickly, taking only three weeks to get from a one-cell embryo to a fully-grown mouse pup. Using a light microscope, we generated this image showing the growth and development of a mouse embryo during the second week of pregnancy. The first embryo is nine days old and has few recognizable features whereas the last embryos is fourteen days old and more closely resembles a mouse pup as birth. Understanding the progression of normal developmental processes will ultimately help us explain the events that cause fetal development to go awry resulting in miscarriage or stillbirth.”
The smallest fetus in the video is the thickness of a penny and the largest one is the size of a blueberry.
More information:
http://www.trophoblast.cam.ac.uk/people/watson.shtml
Department of Physiology, Development and Neurosciences:
http://www.pdn.cam.ac.uk/
Graduate School of Life Sciences:
http://www.biomed.cam.ac.uk/gradschool
Music by Peter Nickalls:
http://www.peternickalls.com
In this video Dr Chris Forman shows us his incredible images of a fruit fly foot and beetle antenna.
Dr Forman:
"Nature has found remarkable ways of using small amounts of energy to combine common elements such as carbon, nitrogen, oxygen and hydrogen into fascinating and complex assemblies like these insects shown here. If we could do the same in our manufacturing processes then perhaps we could recycle our products more easily and we wouldn't use as much energy processing large lumps of aluminium, concrete and plastic. My research into biomaterials tries to learn from nature how to combine the same basic materials into a wide range of structures that perhaps, one day, may be used in all kinds of products from food to factories!"
Size of these images:
μm = micron (one thousandth of a millimetre).
Fruit fly foot: 50 μm across (thin strand of human hair).
Antenna: 60-70 μm across (strand of human hair).
More info about this research:
http://www.cam.ac.uk/research/?p=24151
More images:
http://flic.kr/s/aHsjwMhSFd
Dr Chris Forman's profile:
http://www.ifm.eng.cam.ac.uk/people/cjf41
Thanks to Dr Bill O'Neill and Dr Paul Barker.
Department of Engineering:
http://www.eng.cam.ac.uk
Music by Joe Snape:
http://www.larmlicht.wordpress.com
Find more Cambridge research here:
http://www.cam.ac.uk/research
In the first of this microscopic video series, Dr Chris Forman shows us the eye of a beetle and the eye of a fruit fly and explains how they have inspired technology.
Dr Forman:
“Nature has found remarkable ways of using small amounts of energy to combine common elements such as carbon, nitrogen, oxygen and hydrogen into fascinating and complex assemblies like these insects shown here. If we could do the same in our manufacturing processes then perhaps we could recycle our products more easily and we wouldn't use as much energy processing large lumps of aluminium, concrete and plastic. My research into biomaterials tries to learn from nature how to combine the same basic materials into a wide range of structures that perhaps, one day, may be used in all kinds of products from food to factories!”
Size of these images:
μm = micron (one thousandth of a millimetre)
Beetle eye: each individual lens is 12 μm (the thickness of cling film), the entire eye is about 750 μm across (thickness of 5 sheets of paper) and the entire image is about 240 μm across (really thick bit of human hair).
Fruit fly eye: Again each lens is about 10 μm (thickness of cling film), and the entire eye is about 200-300 μm (3 sheets of paper). The total distance across the image is about 115 μm across (thickness of a human hair).
More info:
cam.ac.uk/research/?p=24151
More images:
flic.kr/s/aHsjwMhSFd
Dr Forman’s profile:
ifm.eng.cam.ac.uk/people/cjf41
Thanks to Dr Bill O’Neill and Dr Paul Barker.
Department of Engineering:
eng.cam.ac.uk
Music by Joe Snape:
larmlicht.wordpress.com
Comments
Top Podcasts
The Best New Comedy Podcast Right Now – June 2024The Best News Podcast Right Now – June 2024The Best New Business Podcast Right Now – June 2024The Best New Sports Podcast Right Now – June 2024The Best New True Crime Podcast Right Now – June 2024The Best New Joe Rogan Experience Podcast Right Now – June 20The Best New Dan Bongino Show Podcast Right Now – June 20The Best New Mark Levin Podcast – June 2024